The IceCube Neutrino Observatory is primarily known for detecting hard-to-catch particles, called neutrinos, using its cubic-kilometer array of light sensors buried under the ice at the geographic South Pole. But IceCube also has a surface component, known as IceTop, that detects cosmic rays—charged particles that reach Earth from outer space at high energies. (Some of the same particles interact near their cosmic sources to produce the astrophysical neutrinos that IceCube sees.)
The IceTop surface array is made up of 81 stations spaced about 125 meters apart spread over a square kilometer at the South Pole. Each station consists of two tanks separated by 10 meters that each house two optical sensors frozen in water. When cosmic rays collide with particles in Earth’s atmosphere, they create secondary showers of particles, called “air showers.” The particles from these air showers are detected by IceTop’s tanks, allowing scientists to reconstruct the original cosmic ray’s energy and direction.
Now, IceCube has found a way to detect cosmic rays of lower energies previously unreachable by IceTop. In a paper submitted to Physical Review D, “Cosmic Ray Spectrum from 250 TeV to 10 PeV using IceTop,” the IceCube Collaboration explains how they implemented a new two-station trigger as well as the machine-learning method developed to analyze these events.
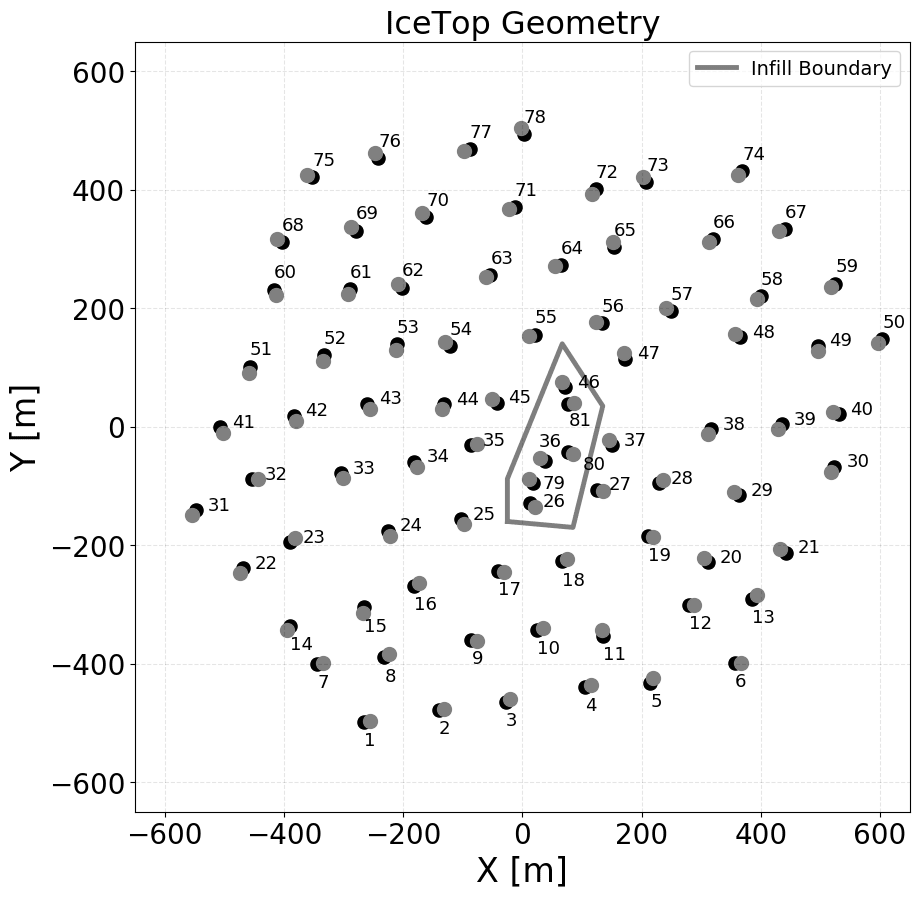
The cosmic-ray energy spectrum is the distribution of cosmic rays based on their energies. Precise measurements of the energy spectrum and elemental composition are necessary to understand cosmic-ray sources, acceleration, and propagation.
The more energetic the cosmic ray, the more IceTop stations its air shower hits. Previously, energy spectrum analyses only used air showers that triggered five or more IceTop stations, which resulted in an energy spectrum ranging from 2 PeV (1015 electronvolts) to a few EeV (1018 electronvolts). To lower the energy threshold and study lower-energy cosmic rays, IceCube scientists must use data from air showers with signals on fewer than five stations.
But three- or four-station events were previously ignored—even though the data were collected—because it was so difficult to reconstruct the cosmic ray’s primary energy and direction of arrival with so few stations. Two-station events were not even collected because they’re even more difficult to reconstruct.
So IceCube collaborator Ramesh Koirala, a PhD student at the University of Delaware at the time, developed a trigger that would collect these two-station events from stations less than 50 meters away from each other. Then, collaborators developed a method to reconstruct their primary energy and direction with reasonable accuracy using a machine-learning technique called random forest regression. Based on reconstructed energies, they calculated that the method lowers the threshold of the energy spectrum from 2 PeV to 250 TeV (1012 electronvolts)—nearly an order of magnitude.
The new method also reduces the gap between IceTop and measurements from instruments like balloons and satellites. Since these instruments detect cosmic rays directly, they can determine the composition of cosmic rays more accurately than IceTop’s indirect measurements.
“One of the reasons to lower the energy threshold is to overlap the IceTop spectrum with energy spectra from direct measurement,” says Koirala, who is now a postdoctoral researcher at Nanjing University in China. “This gives us an extra handle in our composition analysis. Also, lowering the energy threshold gives IceTop the full coverage of the ‘knee’ region, which is one of the major features of the cosmic-ray energy spectrum.”
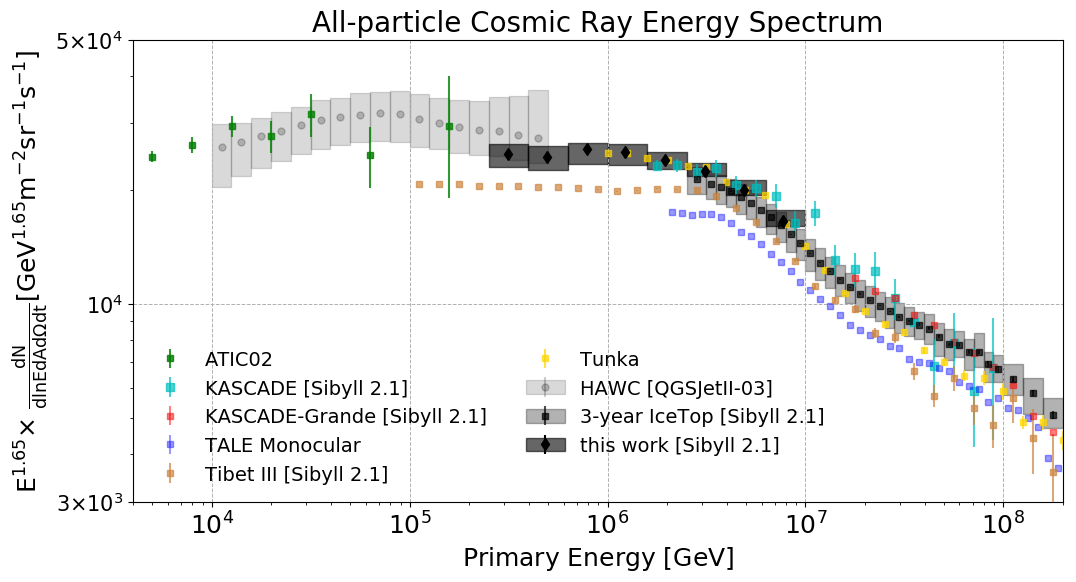
This analysis is the first to make use of the closely spaced detectors to extend the measurement of the cosmic-ray energy spectrum.
“The next step for the future will be to use events that pass the two-station trigger on the surface and that also have signals in the array of IceCube DOMs two kilometers below the surface,” says Thomas Gaisser, an emeritus professor at the University of Delaware and another lead on this paper. The ratio of the deep signal to the surface signal will give scientists information about the nuclear composition of those primary cosmic-ray particles that initiate the cascades detected by IceTop.
info “Cosmic Ray Spectrum from 250 TeV to 10 PeV using IceTop,” IceCube Collaboration: M. G. Aartsen et al., Physical Review D 102 (2020) 122001, journals.aps.org, arxiv.org/abs/2006.05215