Detector
IceCube, the South Pole neutrino observatory, is a cubic-kilometer particle detector made of Antarctic ice and located near the Amundsen-Scott South Pole Station. It is buried beneath the surface, extending to a depth of about 2,500 meters. A surface array, IceTop, and a denser inner subdetector, DeepCore, significantly enhance the capabilities of the observatory, making it a multipurpose facility.
IceCube is the first gigaton neutrino detector ever built and was primarily designed to observe neutrinos from the most violent astrophysical sources in our universe. Neutrinos, almost massless particles with no electric charge, can travel from their sources to Earth with essentially no attenuation and no deflection by magnetic fields.
The in-ice component of IceCube consists of 5,160 digital optical modules (DOMs), each with a ten-inch photomultiplier tube and associated electronics. The DOMs are attached to vertical “strings,” frozen into 86 boreholes, and arrayed over a cubic kilometer from 1,450 meters to 2,450 meters depth. The strings are deployed on a hexagonal grid with 125 meters spacing and hold 60 DOMs each. The vertical separation of the DOMs is 17 meters.
Eight of these strings at the center of the array were deployed more compactly, with a horizontal separation of about 70 meters and a vertical DOM spacing of 7 meters. This denser configuration forms the DeepCore subdetector, which lowers the neutrino energy threshold to about 10 GeV, creating the opportunity to study neutrino oscillations.
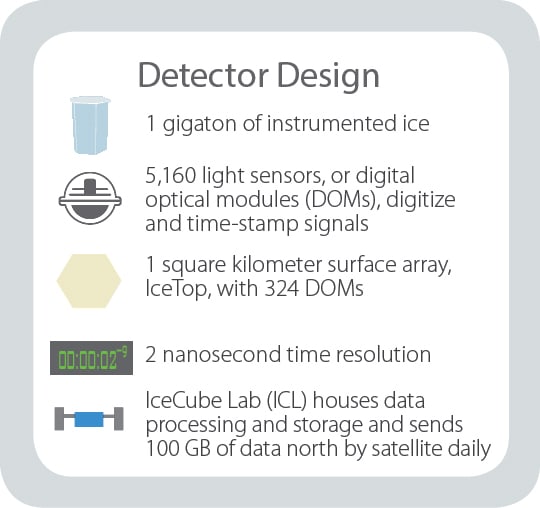
IceTop consists of 81 stations located on top of the same number of IceCube strings. Each station has two tanks, each equipped with two downward facing DOMs. IceTop, built as a veto and calibration detector for IceCube, also detects air showers from primary cosmic rays in the 300 TeV to 1 EeV energy range. The surface array measures the cosmic-ray arrival directions in the Southern Hemisphere as well as the flux and composition of cosmic rays.
Developments in neutrino astronomy have been driven by the search for the sources of cosmic rays, leading at an early stage to the concept of a cubic-kilometer neutrino detector. Cosmic rays, which consist mainly of protons, are the highest energy particles ever observed, with energies over a million times those reached by today’s particle accelerators on Earth.
AMANDA, the Antarctic Muon and Neutrino Detector Array, was built as a proof of concept in the mid 1990s and demonstrated that the extremely clear Antarctic ice was suitable for detecting energetic neutrinos. IceCube, the only cubic-kilometer neutrino detector constructed to date, was completed in December 2010, only six years after the deployment of the first string at the South Pole.
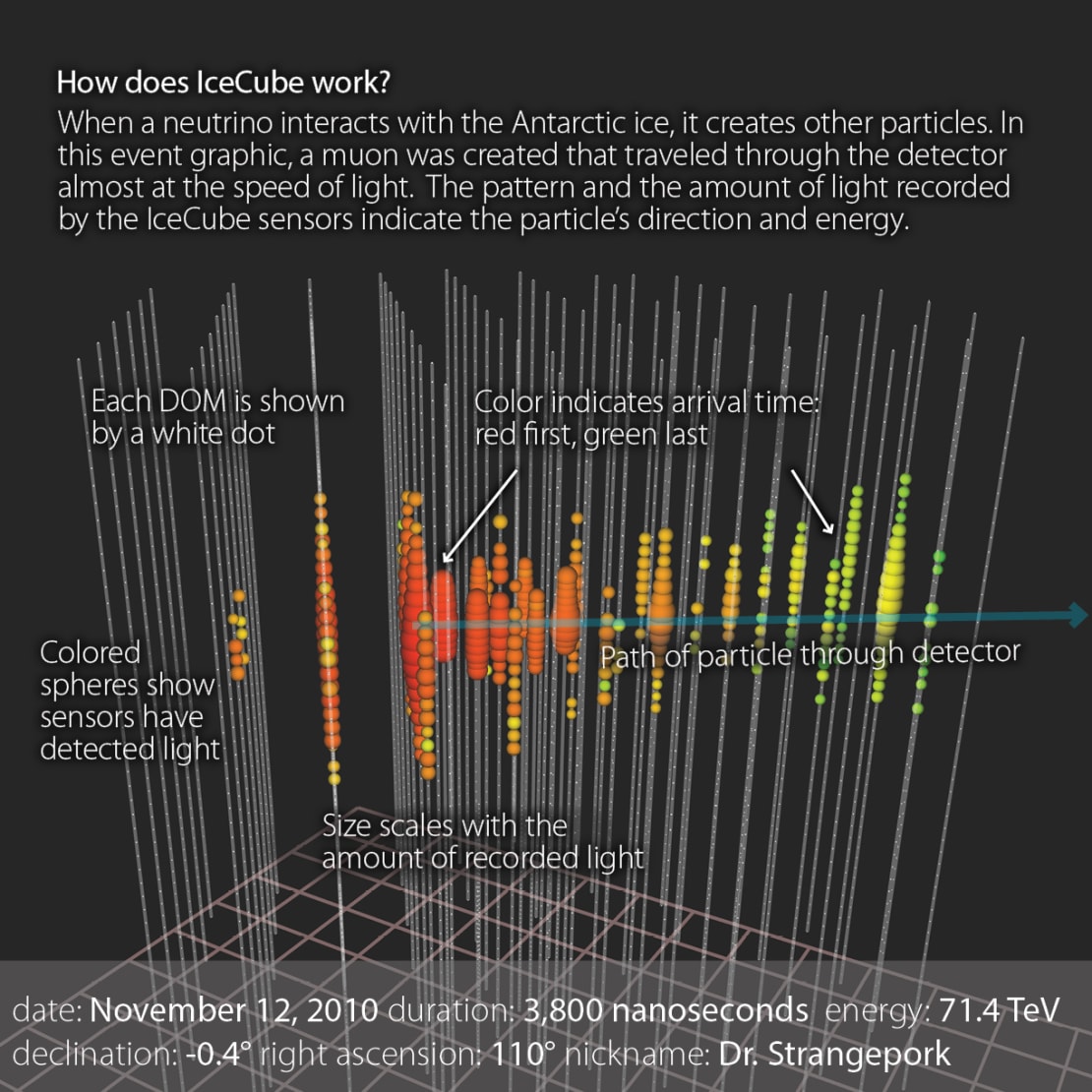
Neutrinos are not observed directly, but when they happen to interact with the ice they produce electrically charged secondary particles that in turn emit Cherenkov light, as a result of traveling through the ice faster than light travels in ice.
The IceCube sensors collect this light, which is subsequently digitized and time stamped. This information is sent to computers in the IceCube Lab on the surface, which converts the messages from individual DOMs into light patterns that reveal the direction and energy of muons and neutrinos.
The IceCube Neutrino Observatory was built under a National Science Foundation (NSF) Major Research Equipment and Facilities Construction grant, with assistance from partner funding agencies around the world. The NSF Office of Polar Programs supports the project with a Maintenance and Operations (M&O) grant. The University of Wisconsin–Madison is the lead institution, coordinating data-taking and M&O activities. The international IceCube Collaboration, with more than 40 institutions worldwide, is responsible for the scientific research program.
Construction
On December 18, 2010, just after 6 pm New Zealand time, the last of IceCube’s 86 strings was lowered into the Antarctic ice. Seven austral summers of construction had come to an end, and IceCube was completed a decade after the collaboration submitted the proposal.
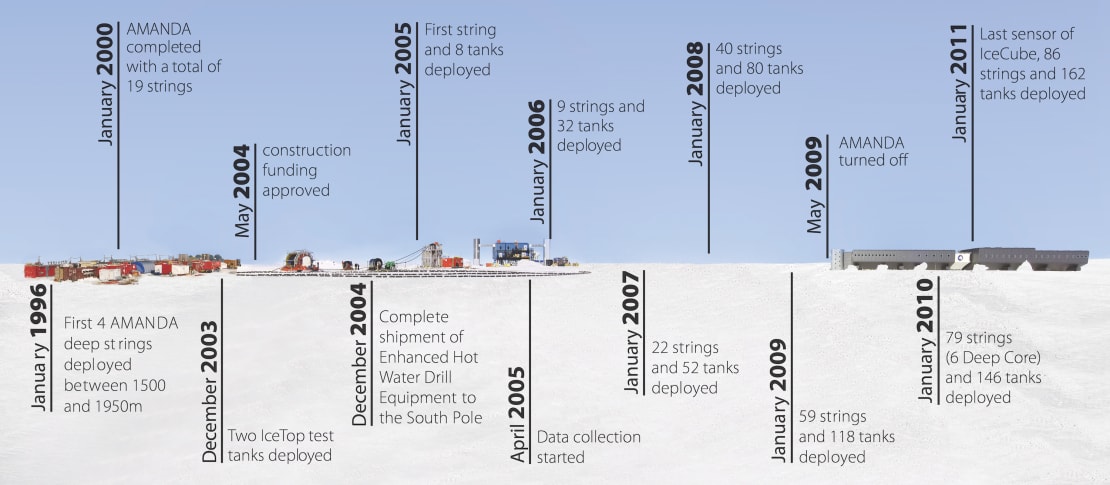
Dedicated teams melted 60-centimeter diameter holes to a depth of 2,450 meters, deployed sensors, and commissioned strings from November to February each year from 2004 until 2011. Deployment specialists spent 11 hours on average to expertly connect sensors to a cable and lower them into the water-filled holes, which eventually refroze. In seven seasons, 86 holes in all were drilled and instrumented.
The drill was designed by the IceCube project and built at the UW Physical Sciences Lab. The high-pressure hose and nozzle delivered hot water that melted through the ice at record speeds, about 2 meters per minute, down to depths of 2,450 meters. A separate drill was designed and deployed to advance through the firn layer, the top 50 meters of compacted snow where hot water drilling is not efficient. Together the two drills were able to consistently produce almost perfect vertical holes ready for deployment of instrumentation at a rate of one hole every two days.
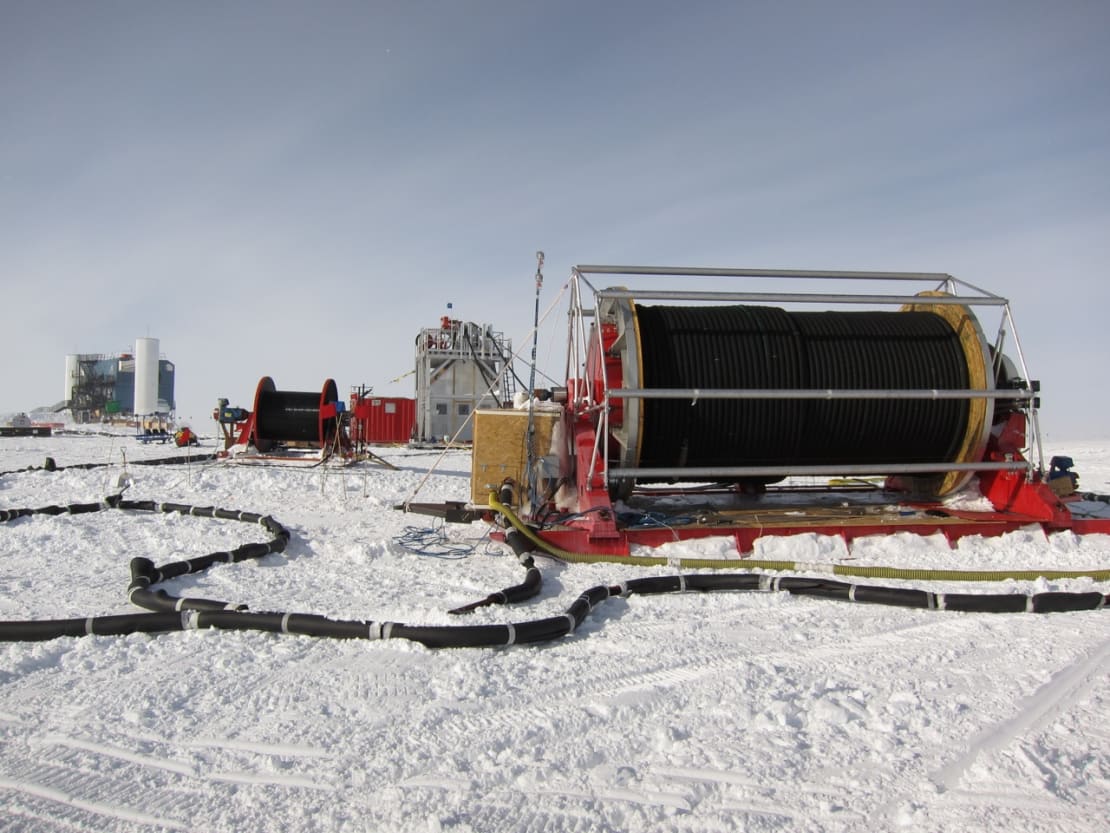
Since water starts to freeze immediately, IceCube sensors were quickly deployed following drilling. Once the refreezing process ended, which took a couple of weeks to stabilize, the failure rate of the instrumentation has been extremely low—fewer than 100 of the approximately 5,500 sensors are currently nonoperational.
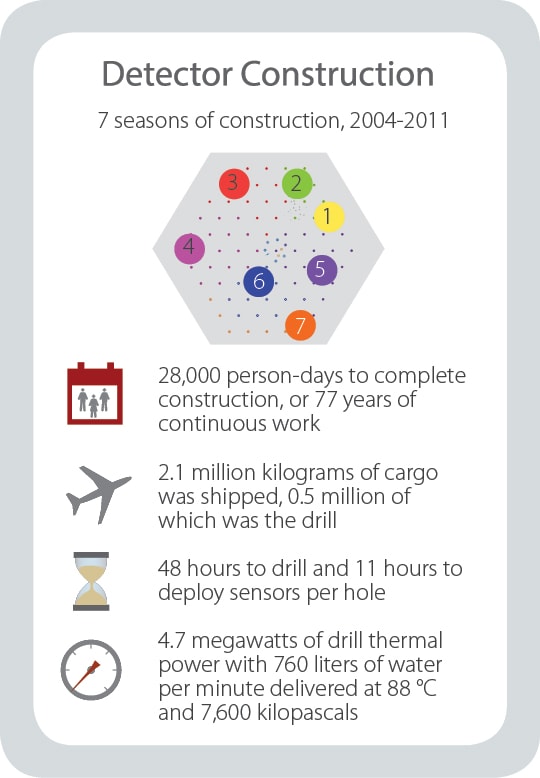
IceCube sensors, designed with main contributions from Lawrence Berkeley National Laboratory, DESY-Zeuthen, and UW–Madison, were produced and tested at the universities in Stockholm and Uppsala in Sweden, DESY-Zeuthen in Germany, and the Physical Sciences Lab in Stoughton, Wisconsin, USA. Each string of sensors had a general theme, with a name given to each of the deployed sensors as a more effective means of identification rather than a numbering system.
Building IceCube was an extraordinary engineering achievement. It was completed on time, on budget, and significantly exceeding performance specifications. This is a tribute to the perseverance and dedication of the physicists, engineers, and technicians that overcame the many challenges of creating and operating a detector in such an inhospitable environment.
The total cost of construction was $279 million. The National Science Foundation provided around $242 million with the remaining funds coming from funding agencies in the US and abroad.