In 2013, the IceCube Neutrino Observatory detected very high energy particles called neutrinos that arrived at Earth from outer space. Not only did IceCube confirm the existence of astrophysical neutrinos, but they also proved that these elusive particles could be detected by their cubic-kilometer-sized telescope buried in the South Pole ice.
Even though IceCube had accomplished one of its main goals only three years into its operation, a mystery remained: Where exactly do astrophysical neutrinos come from? In 2018, IceCube found compelling evidence that blazar TXS 0506+056 was a source of high-energy neutrinos and, more recently, the starburst galaxy NGC 1068 was found to be the “hottest” source in the northern sky. Yet we still don’t know what source classes are capable of generating astrophysical neutrinos and whether there is any particular source class dominating the astrophysical neutrinos that IceCube detects.
In a paper recently submitted to Physical Review Letters, the IceCube Collaboration explored whether IceCube’s neutrinos were coming from the cores of active galactic nuclei (AGN): supermassive black holes at the centers of galaxies that emit enormous amounts of energy. When they searched for neutrino emission from a catalog of over 30,000 sources, the collaboration found an excess of neutrinos coming from the cores of luminous AGN at a significance of 2.6 sigma. This suggests that AGN may be responsible for many of the highest energy neutrinos detected by IceCube—the first time IceCube has had a hint of one population as a major contributor to the astrophysical neutrino flux.
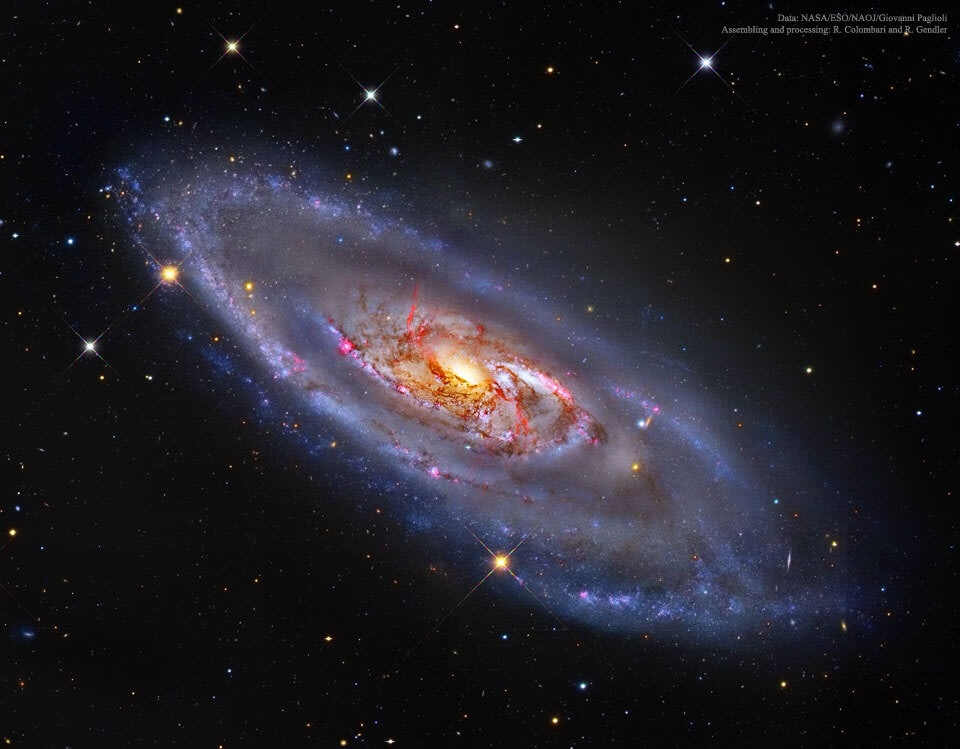
AGN are among the most powerful emitters of radiation in the known universe and are therefore very promising potential sites for high-energy neutrino production. “AGN have the potential to accelerate protons up to the highest observed cosmic-ray energies and are surrounded by high-intensity radiation fields where neutrinos can be produced,” says Federica Bradascio, a postdoc at IRFU CEA-Saclay who led this analysis as a PhD student at DESY.
Despite being powered by supermassive black holes, AGN are incredibly bright due to their accretion disks: the gas and dust that orbit the black hole and emit heat and light. Sometimes, this material gets ejected as relativistic jets, which is one possible mechanism for AGN neutrino production. But IceCube has already explored blazars (a type of AGN with jets) and showed that they can only contribute up to 27% of the astrophysical neutrino flux. So Bradascio and her collaborators decided to focus instead on the accretion disks of AGN.
With eight years of IceCube data from the Northern Hemisphere, the researchers performed a “stacking analysis” that searches for the clustering of neutrinos around the directions of AGN accretion disks. They used three AGN samples combining radio, infrared, and X-ray information to probe two different scenarios of high-energy neutrino production in AGN cores: whether there was neutrino production in the cores of luminous AGN and whether there was neutrino production in the cores of low-luminosity AGN. The three samples contained roughly 10,000 sources each, making it the largest number of sources ever used in a stacking analysis for IceCube.
In a stacking analysis, each source is assigned a “weight” that tells researchers how likely neutrinos are to be produced in that source. Bradascio and her collaborators assigned weights based on each accretion disk’s X-ray flux. “Since we are looking at neutrino production in the accretion disk, which emits X-rays, we can assume that the neutrino flux produced in the accretion disk of a given source is equivalent to the X-ray flux we measure from it,” she explains.
The collaborators found that, for the largest sample (the 32,249 infrared-selected AGN sources), there is an excess of 105 ± 44 neutrinos above expectations from the background, corresponding to a significance of 2.6 sigma. “If we interpret this as a genuine signal, and we assume that there is a proportionality of X-ray and neutrino fluxes as we hypothesized, this observation implies that at 100 TeV, 27% to 100% of the observed neutrinos arise from particle acceleration in the core of AGN,” says Bradascio. “These findings represent the first direct hint that cosmic rays accelerated in the AGN core regions are responsible for the bulk of the astrophysical neutrino flux observed by IceCube above 100 TeV at about 2.6 sigma significance.”
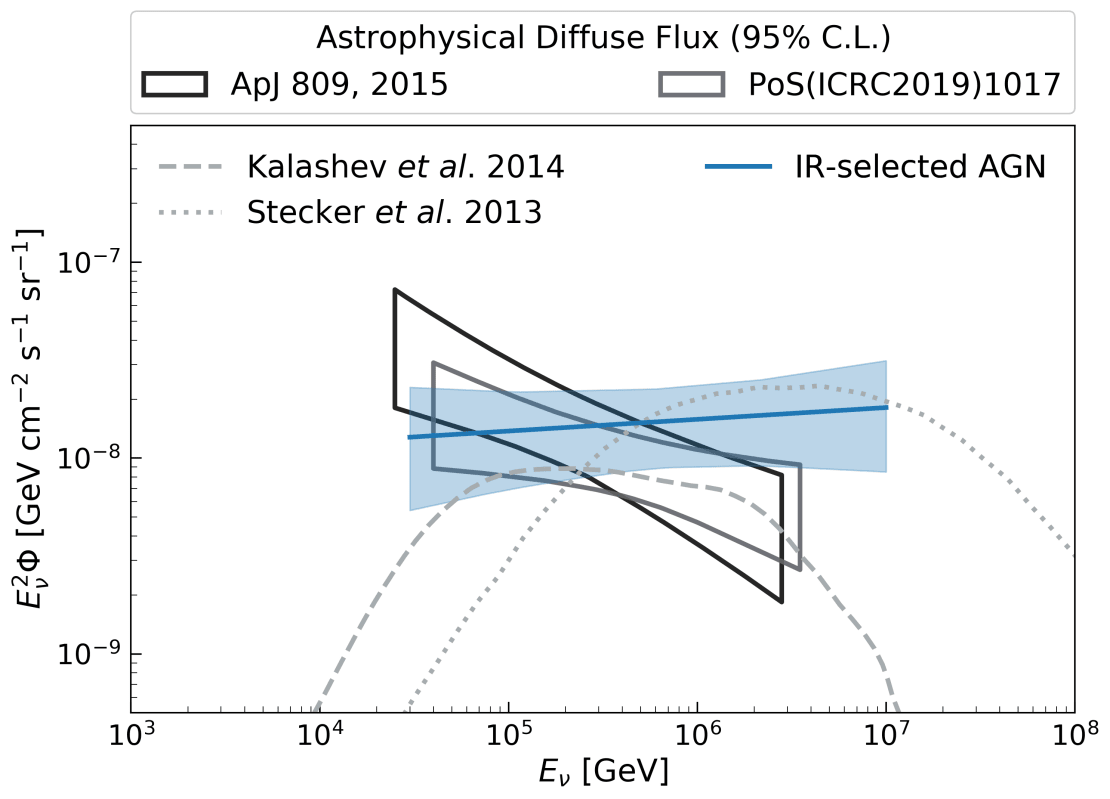
The results also suggest that the AGN population is opaque to high-energy gamma rays since gamma rays are expected to be absorbed in these dense environments. This means that neutrinos are more likely to be produced in sources that are hidden in gamma rays, which will inform future analyses. It also means that gamma-ray sources cannot explain the origins of all of the IceCube flux, confirming previous results by Fermi-LAT and IceCube.
In the future, Bradascio says researchers can conduct follow-up analyses by using significantly larger IceCube datasets and better angular resolutions, employing the proposed next-generation detector, IceCube-Gen2, and compiling deeper AGN catalogs using, for example, new X-ray surveys.
“It is the first time that a particular population has been found to contribute so much to the IceCube flux,” says Bradascio. “It is therefore essential to follow up on these initial indications in the future.”
+ info “A search for neutrino emission from cores of Active Galactic Nuclei,” The IceCube Collaboration: R. Abbasi et al. Submitted to Physical Review Letters arxiv.org/abs/2111.10169