We are all surrounded by magnets. Whether they’re on your fridge, in your smartphone, or in your credit card, the magnets you interact with all have one thing in common (well, two things): they each have a north and south pole.
Could a magnet ever have just one pole? Yes, according to scientists, and it’s called a magnetic monopole. But no one has ever seen one.
The Standard Model of particle physics, the rulebook that tells us how the universe works at the smallest scales, allows for the existence of magnetic monopoles. In fact, magnetic monopoles should have been produced in the early universe, and since they are stable, there should be a relic flux of them leftover from the Big Bang still permeating all of space. Yet they have never been detected.
An enormous neutrino detector at the South Pole might be able to help. If magnetic monopoles exist, they should pass through the IceCube Neutrino Observatory, leaving behind a unique pattern. So IceCube researchers recently performed a search for magnetic monopoles with speeds close to the speed of light. They did not find evidence of magnetic monopoles after analyzing eight years of IceCube data, and their results are presented in a paper recently accepted by Physical Review Letters.
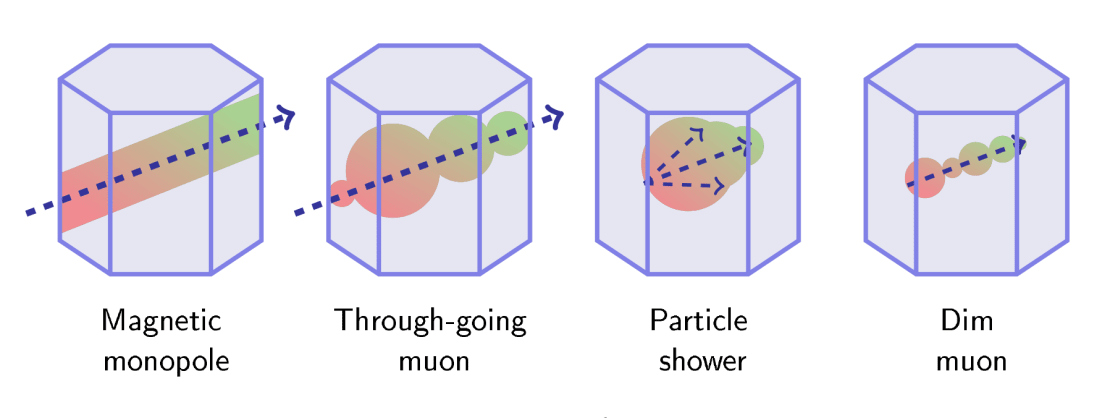
The IceCube Neutrino Observatory is a strange telescope. Made up of over 5,000 light sensors buried in a cubic kilometer of ice at the South Pole, its original goal was to study neutrinos—lightweight fundamental particles that pervade the universe—even though it can’t actually see them. Neutrinos are so elusive that the only way to study them is by analyzing the products of their rare interactions with matter, like studying an animal based on the tracks it leaves behind in the snow.
If a neutrino collides with an atom in the ice around IceCube, it can produce a charged particle called a muon. When a muon travels fast enough through the ice, it generates a cone of blue radiation along its path called Cherenkov light. This light travels through the ice, triggering IceCube’s sensors as it goes, leaving a track of activated sensors that tells IceCube scientists the particle’s energy and direction.
Magnetic monopoles traveling at close to the speed of light also emit Cherenkov light when passing through the IceCube detector. But something sets them apart: They are very bright. Magnetic monopoles emit about 8,000 times more Cherenkov light than muons, and, unlike muons, the light emission is uniformly distributed along their path. This results in a very distinct signature in IceCube.
So IceCube researchers decided to search eight years of IceCube data for signs of magnetic monopoles. They first selected very bright events and looked for uniform emission along the path. Since magnetic monopoles can cross the detector entirely, they were able to eliminate tracks that started or stopped within the volume of IceCube. Researchers then trained a machine learning event characterization tool (a boosted decision tree) to differentiate magnetic monopole events from neutrino and muon events in the sample.
Unfortunately, the search did not yield any signature of cosmic magnetic monopoles. “But negative results in science also have a positive spin. They teach us about what is not happening in nature, which is as important as understanding what is happening,” says Carlos Pérez de los Heros, a professor at Uppsala University in Sweden who supervised the analysis. “In this case we have set a very strong limit—world leading—on the flux of relativistic cosmic monopoles: their flux must be below a certain value, otherwise we would have detected them.” This limit, which is 10 times stricter than the previous limit, has implications on models of the evolution of the early universe and how the balance between matter and antimatter developed as the universe evolved.
Looking forward, scientists will continue to search for a relic flux of magnetic monopoles with neutrino telescopes and other detectors. “IceCube is uniquely suited for this type of analysis, so the present result will probably remain world-leading until enough new data is collected to significantly supersede it,” says Alexander Burgman, who led the analysis as a PhD student at Uppsala University and is now a postdoctoral researcher at Lund University. He and Pérez de los Heros point out that the proposed expansion of IceCube, IceCube-Gen2, would significantly speed up the search; Gen2’s 10-times-larger volume would allow collaborators to increase the sensitivity to this relic flux of magnetic monopoles.
For now, the mystery of the missing magnetic monopoles persists. But that doesn’t mean they don’t exist. “Magnetic monopoles are ‘guaranteed’ particles in our favorite models of the early universe,” says Pérez de los Heros, “and if indeed they do not exist, we must reconsider fundamental assumptions of our current world model.”
+ info “Search for Relativistic Monopoles with Eight Years of IceCube Data,” IceCube Collaboration: R. Abbasi et al., Physical Review Letters 128 (2022) 5, 051101, journals.aps.org, arxiv.org/abs/2109.13719