Many people think of our sun as being bright. It is an enormous ball of glowing gas that illuminates our days and provides Earth with heat and light. But it is also being explored for signals of something darker: dark matter.
Dark matter is not literally dark; it is invisible because it does not interact with light or forces besides gravity. But it is called “dark” because scientists know so little about it. The mysterious substance makes up over 80% of all matter in the universe, and yet it is a major open mystery in particle astrophysics. Physicists have a few ideas about what it could be, including some candidate particles like the weakly interacting massive particle, or WIMP. These hypothetical particles are pulled into the sun gravitationally where they accumulate until their density builds up and they annihilate into Standard Model particles—including neutrinos.
Therefore, if WIMPs are annihilating inside the sun, we should be able to detect an excess of neutrinos coming from it. That is what the IceCube Collaboration looked for using seven years of IceCube data for a paper recently submitted to Physical Review D. Though they did not find a significant detection of neutrinos from the sun, the researchers set constraints on the annihilation of dark matter into neutrinos, the strictest so far among indirect dark matter search experiments.
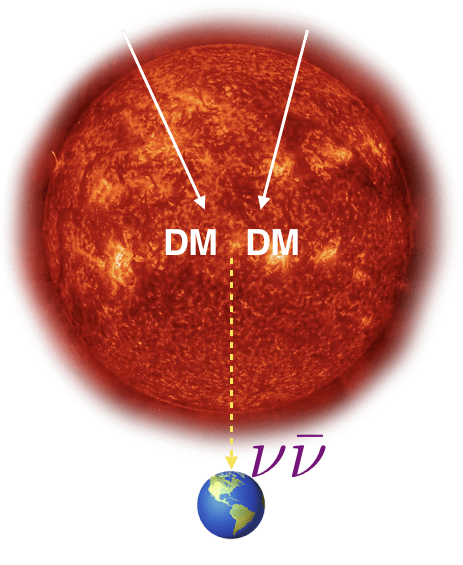
Because dark matter interacts so rarely with normal matter, finding it would require exceedingly large detectors. But that can be costly and impractical. “Instead of building a large detector here on Earth to directly study dark matter, we can indirectly study how it interacts in other astrophysical bodies such as the sun,” says Mehr Un Nisa, a postdoctoral researcher at Michigan State University and lead on this analysis. “IceCube is in a good position to do that by analyzing neutrinos correlated with the direction of the sun.”
The IceCube Neutrino Observatory is an enormous array of ultrasensitive light sensors buried in the ice at the South Pole. When neutrinos interact with atoms in the ice close to IceCube, they produce a shower of charged particles that, when traveling fast enough, emit faint light called Cherenkov radiation. This radiation is picked up by IceCube’s sensors and converted to data that tell researchers about the energy and direction of the original neutrino.
IceCube collaborators can therefore conduct searches that look for neutrinos coming from a specific part of the sky—in this case, the sun. If WIMPs do exist and self-annihilate in the sun, the resulting neutrinos are the only particles that can escape our star’s high-density interior and make it to Earth. “By looking for neutrinos from the sun with a specific energy spectrum, we can comment on the underlying physics of dark matter interactions in the sun,” explains Nisa.
For this search, Nisa and her collaborators focused on looking for low-energy neutrinos: neutrinos with energies less than 100 GeV (GeV = 109 eV). Because of this, they focused on data from IceCube’s inner subset of sensors known as DeepCore; this infill is denser than the rest of IceCube, making it particularly suited for detecting lower energy neutrinos. Since IceCube is optimally sensitive to high-energy neutrinos (1012–1015 eV), the GeV energy frontier has not been extensively explored until now.
After analyzing seven years of DeepCore data, the researchers did not detect any significant excesses of neutrinos from the sun. However, they were able to place very strict constraints on how often dark matter annihilates within the sun, which is determined by how strongly it interacts with protons in the sun. “In the absence of a clear detection,” says Nisa, “the field moves forward by excluding certain values of dark matter masses and their interactions with matter, allowing theorists to focus their efforts on new models.”
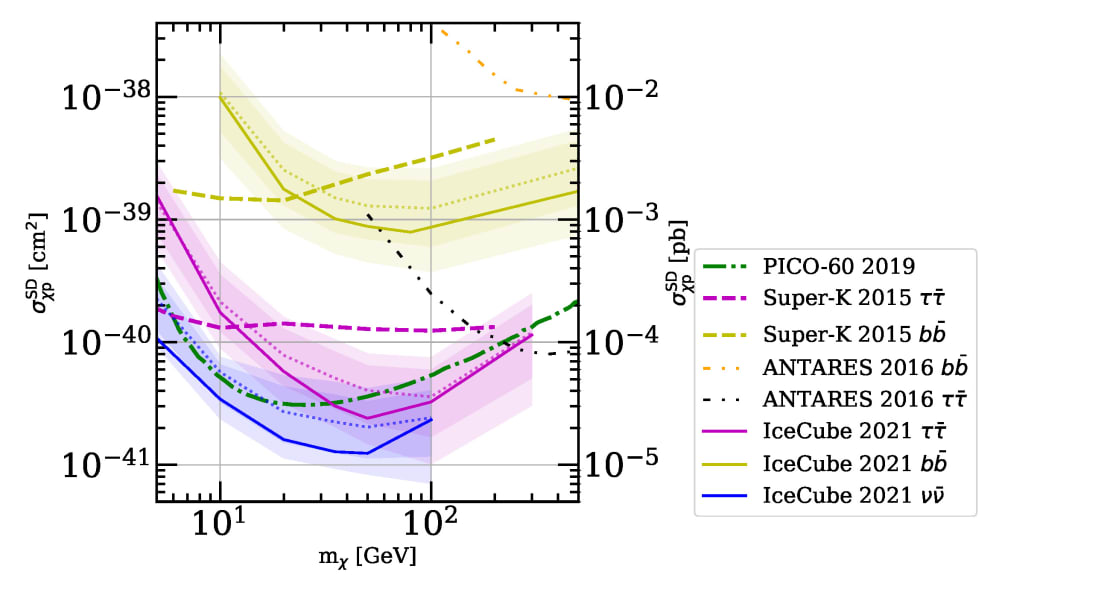
On the—ahem—bright side, Nisa says the sun is just one of many targets for indirect dark matter searches in IceCube. In the future, she says IceCube can continue improved searches for signatures of dark matter annihilation from other targets in the sky, such as dwarf galaxies, galaxy clusters, the Galactic Center, and even Earth. Future and ongoing work will continue to explore these targets with improved sensitivity to a wider range of dark matter masses.
+ info “Search for GeV-scale Dark Matter Annihilation in the Sun with IceCube DeepCore,” IceCube Collaboration: R. Abbasi et al., Physical Review D 105 (2022) 6, 062004, journals.aps.org, arxiv.org/abs/2111.09970