The IceCube Neutrino Observatory is an unusual telescope. It uses its over-5,000 optical sensors embedded in the South Pole ice to look for signals from astrophysical neutrinos, nearly massless subatomic particles from outer space. One of IceCube’s main goals is to pinpoint sources of these neutrinos, of which there are many.
Since a 2013 IceCube paper showed the first solid evidence for astrophysical neutrinos from cosmic accelerators, we have learned some things about the sources of these mysterious particles. In 2017, IceCube detected a neutrino coming from a blazar called TXS 0506+056, the first compelling evidence of an astrophysical neutrino source. And a recent analysis of 10 years of IceCube data singled out another potential source: the Seyfert II galaxy NGC 1068, a type of active galactic nuclei.
However, sources of most astrophysical neutrinos remain unknown, and the IceCube Collaboration continues to search for them using a variety of analysis methods. In a paper submitted yesterday to The Astrophysical Journal, the collaboration describes a time-dependent all-sky scan using five years of IceCube data as well as a specific analysis of blazar 3C 279. The method involves looking for neutrinos clustered in both time and space—in other words, neutrinos that came from the same spot in the sky and arrived within a certain period. This analysis, which is an update of a previous time-dependent all-sky scan from 2015, did not reveal any new neutrino point sources.
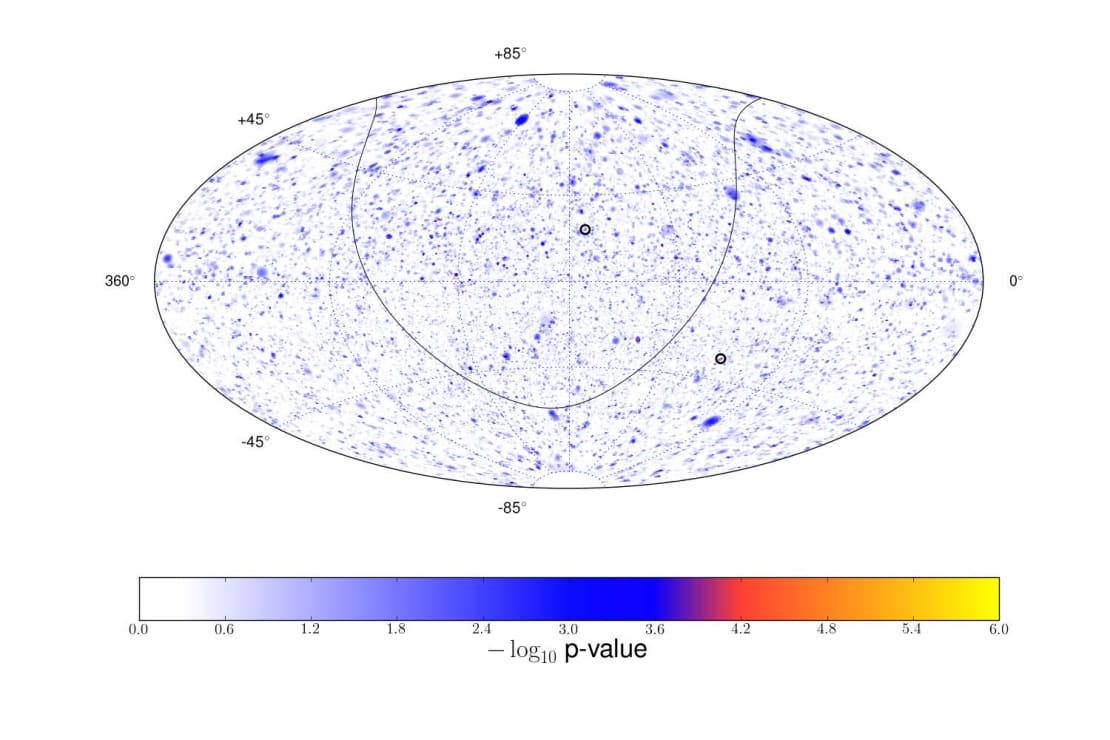
We don’t know a lot about neutrino sources, which makes it a challenge to look for them. IceCube researchers perform many different searches under different hypotheses, including time-integrated searches (used to find the potential source NGC 1068) and time-dependent searches. Unlike time-integrated searches, time-dependent searches consider the neutrinos’ times of arrival at IceCube, focusing on neutrinos clustered in both space and time. Time-dependent searches have a better discovery potential than time-integrated searches for a potential continuous emission of neutrino signal that lasts less than 100 days. Plus, searching for neutrinos that cluster both spatially and temporally helps further separate potential neutrino signals from background, as researchers expect the background flux of neutrinos to be constant in time.
IceCube researchers took the time-dependent approach for the all-sky scan of five years of IceCube data. “We developed this likelihood method over the years starting with my time at the University of Wisconsin–Madison,” says Teresa Montaruli, now a professor in the particle physics department at the University of Geneva in Switzerland.
“We don’t assume anything about the source, but only search for clustering of astrophysical neutrinos in space and time over the whole sky,” says Stéphanie Bron, a PhD student at the University of Geneva and a lead on this analysis.
Montaruli, Bron, and their colleagues examined the sky in 0.1-by-0.1 degree squares and calculated whether neutrino data in each square was compatible with what they expected for background. The point found to be least compatible with background in each hemisphere was deemed the “hottest spot.”
Over the five years that the researchers scanned, no new astrophysical neutrino point source was found. The most significant hotspot was compatible with background fluctuation and was not associated with a known gamma-ray source. However, the second-most significant hotspot was compatible with the location of TXS 0506+056, the blazar from the 2017 detection, though it was not a significant result in this analysis. Bron says this discrepancy is because they did not focus the scan on this part of the sky. “Because we are not looking only in the direction of TXS, but scanning the whole sky, we are penalized by the huge trial factor, which greatly reduces the final significance of the result,” she says. “It is interesting to see in practice the kind of effect that those trial factors might have, and this really points to the importance of using multimessenger signals to drive the search for cosmic neutrinos.”
The researchers also tried their method in a more focused search. With measurements from the Fermi-LAT gamma-ray telescope, they targeted blazar 3C 279, which underwent an exceptionally bright flare on June 16, 2015. Seeing gamma rays and neutrino emission from the same source would be a strong indication of a cosmic ray source, so this blazar was an ideal object to examine.
For this part of the analysis, researchers only looked at the sky in the direction of the blazar and only analyzed eleven days of IceCube data centered on the day of the flare. Alas, the analysis revealed no significant results. However, the researchers were able to establish an upper limit to constrain a model of the number of neutrino events associated with this specific flare of 3C 279 that IceCube should detect under certain assumptions about the gamma-ray emission observed by Fermi-LAT.
Still, Montaruli, Bron, and their colleagues believe that this type of time-dependent analysis should continue. “Even though the analyzed data sets didn’t lead to any significant results, a future data set might,” says Bron. “The nice thing about this kind of analysis is that it doesn’t depend on any assumption about the shape, duration, or source of the signal. So it is sensitive to a whole range of phenomena, including gamma-ray bursts and active galactic nuclei, but also phenomena that maybe we don’t even know exist. And this is the exciting part to me!”
info “A Search for Time-depende 911 (2021) 1, 67t Astrophysical Neutrino Emission with IceCube Data from 2012 to 2017,” IceCube Collaboration: R. Abbasi et al., The Astrophysical Journal, iopscience.iop.org, arxiv.org/abs/2012.01079