Like ice cream, particles come in different flavors. According to the particle physics rulebook, known as the “Standard Model,” an extremely light fundamental particle called the neutrino comes in three: electron, muon, and tau. But there’s a twist—a neutrino can change, or “oscillate,” between its three flavors as it travels through space. It’s as if you picked up a chocolate ice cream cone, but it was vanilla by the time it reached your mouth.
In the last three decades, however, several neutrino oscillation experiments have reported results that don’t comply with this picture. One explanation for the anomalous measurements is the existence of a fourth type of neutrino called the sterile neutrino. If it exists and has a particular mass (on the scale of an electronvolt, or eV), a sterile neutrino should produce a unique feature that is detectable by the IceCube Neutrino Observatory.
In two new papers, the IceCube Collaboration updates their eV-scale sterile neutrino search using an eight-year dataset and improved event selection. The analysis found no evidence of sterile neutrinos at this energy scale and was consistent with the no-sterile-neutrino hypothesis. “An eV-Scale Sterile Neutrino Search Using Eight Years of Atmospheric Muon Neutrino Data from the IceCube Neutrino Observatory” was submitted to Physical Review Letters, and its companion paper, “Searching for eV-Scale Sterile Neutrinos with Eight Years of Atmospheric Neutrinos at the IceCube Neutrino Telescope,” was submitted to Physical Review D.
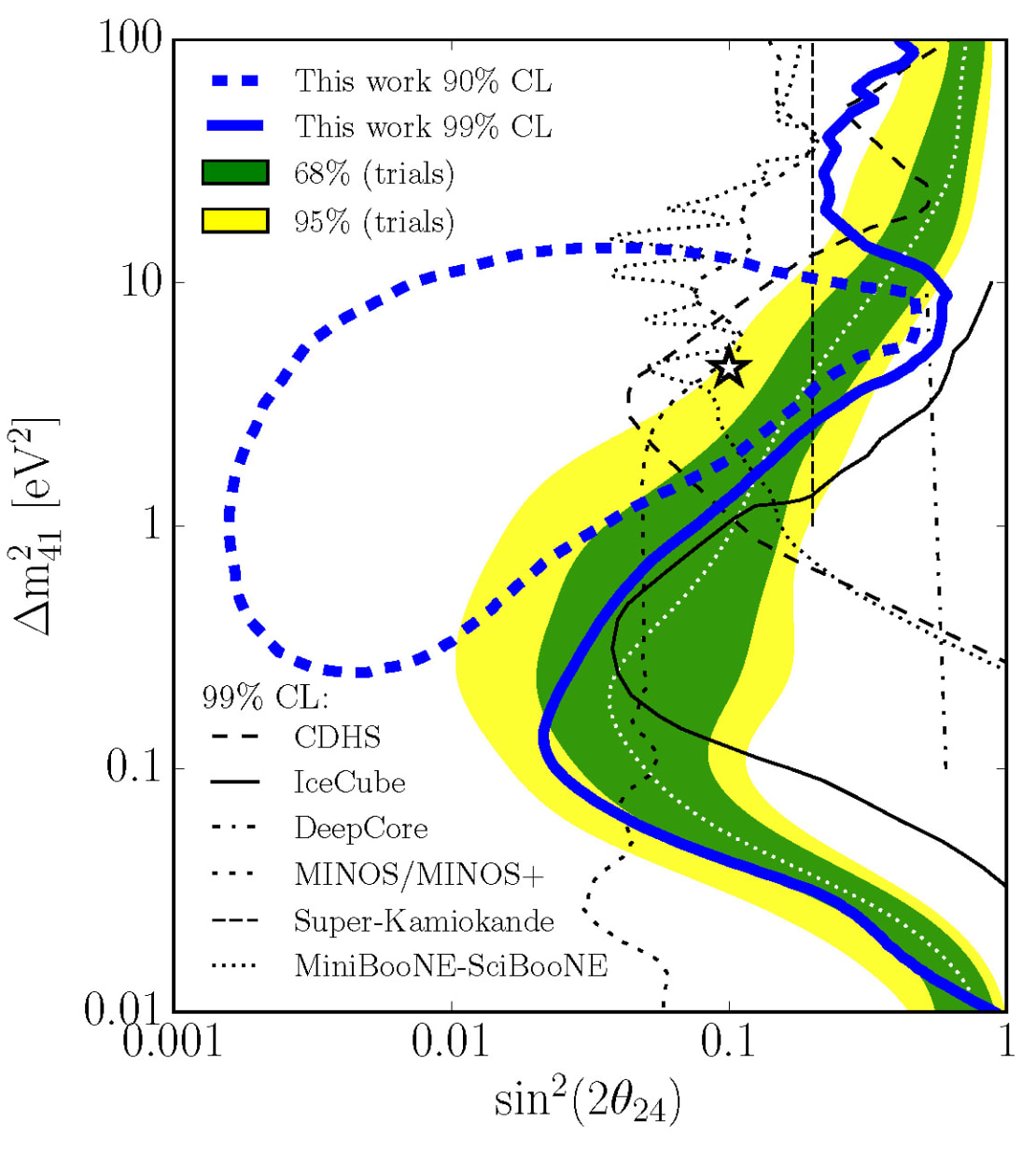
Neutrinos are hard to catch. Even though they are extremely abundant—over a thousand trillion will pass through your body as you read this sentence—they are so weakly interacting that they pass through many light-years of matter without betraying any sign of their presence.
At the South Pole, the IceCube Neutrino Observatory acts as a fishing net for these elusive particles. Over 5,000 light sensors, which are arranged in a cubic-kilometer grid a mile below the surface of the ice, are on the lookout for signals caused by particles created when high-energy neutrinos collide with atoms in the ice. But since the theorized sterile neutrinos do not interact like other neutrinos do, IceCube cannot observe them the same way.
However, IceCube can observe a unique physical phenomenon known as “matter-enhanced resonance” that is predicted by the sterile neutrino hypothesis.
When matter-enhanced resonance occurs, it results in the near-complete disappearance of muon neutrinos of a certain energy (on the scale of a trillion electronvolts, or TeV) passing through Earth’s core. So as IceCube records the number of neutrinos with different energies coming from the direction of Earth’s core, IceCube collaborators look for a dip in the number of TeV-level neutrinos. That would be smoking-gun evidence for a sterile neutrino.
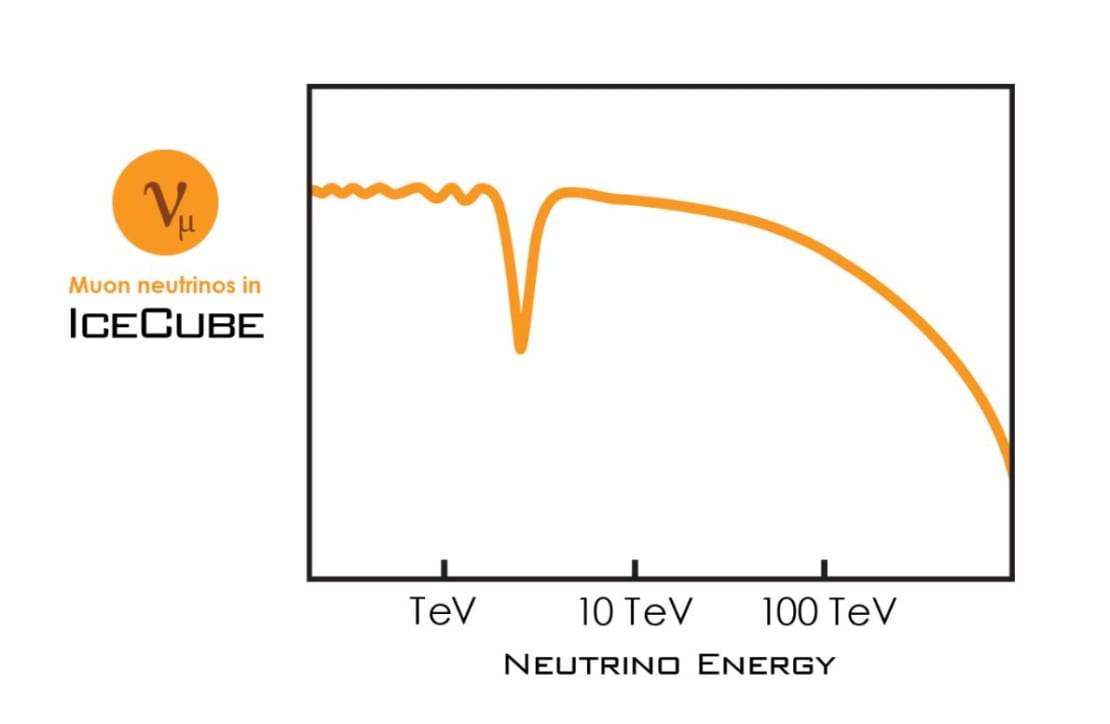
And that’s what IceCube collaborators looked for using eight years of calibrated IceCube data. They measured the direction and energy of over 305,000 atmospheric and astrophysical muon neutrinos. Then, they compared their data to predictions for various sterile neutrino hypotheses while also incorporating 18 different systematic uncertainties. This analysis improved upon a 2016 IceCube analysis that looked for the same feature in just one year of IceCube data.
“This new analysis is very exciting as it has about fifteen times more data than our previous analysis,” says Carlos A. Argüelles, postdoctoral researcher at the Massachusetts Institute of Technology and a lead on this analysis. “In the last three years we have been engaged with the development of an updated systematic treatment appropriate for this very large data set.”
The researchers found no evidence of an eV-scale sterile neutrino in eight years of IceCube data. When compared to the sterile neutrino parameter space—the combination of a variety of physical properties that the sterile neutrino might have—the analysis’s “best-fit” point is consistent with the no-sterile-neutrino hypothesis at a p-value of eight percent. In other words, the data show no significant evidence for the existence of an eV-scale sterile neutrino.
“We are very pleased to be able to share these new results with the world, which have been over three years in the making,” says Ben Jones, assistant professor of physics at the University of Texas at Arlington and a lead on this analysis. “The unique sample of high-energy neutrinos detected by IceCube has given us unprecedented power to search for these exotic but elusive new particles—sterile neutrinos.”
“This result is interesting because we searched for a unique signature of the sterile neutrino prediction—one that only IceCube is capable of measuring at this moment in time,” says Spencer Axani, a graduate student at MIT and another lead on this analysis. “Our analysis provides some of the world’s leading limits on interesting areas of the sterile neutrino parameter space.”
It now appears that an eV-scale sterile neutrino is no longer one of these interesting areas, but there are more areas of the parameter space yet to be explored. The sterile neutrino may still be out there, and if found may reveal how neutrinos acquire their tiny masses.
info “An eV-scale sterile neutrino search using eight years of atmospheric muon neutrino data from the IceCube Neutrino Observatory,” The IceCube Collaboration: M. G. Aartsen et al. Submitted to Physical Review Letters arxiv.org/abs/2005.12942
info “Searching for eV-scale sterile neutrinos with eight years of atmospheric neutrinos at the IceCube neutrino telescope,” IceCube Collaboration: M. G. Aartsen et al., Physical Review D 102 (2020) 5, 052009, journals.aps.org, arxiv.org/abs/2005.12943