Neutrinos are nearly massless and weakly interacting fundamental particles that can travel millions of light-years unperturbed. They come in three types, called flavors: electron, muon, and tau. Some neutrinos originate on Earth in nuclear reactors, in the center of our sun, and when cosmic particles crash into the atmosphere. But others are born in cosmic events like exploding stars or in the vicinity of black holes of active galaxies.
Highly energetic neutrinos that come from beyond our solar system are known as astrophysical neutrinos. They are the main focus of study for the IceCube Neutrino Observatory at the South Pole; in fact, identifying astrophysical sources (aka “cosmic accelerators”) of these particles is one of the scientific goals of IceCube.
In 2013, IceCube discovered neutrinos of extragalactic origin. A measurement of the intensity of such a neutrino stream is known as a neutrino flux. In a paper recently submitted to Physical Review Letters, the IceCube Collaboration presents new results of an analysis to determine and characterize, with unprecedented precision and across the entire sky, the combined flux of astrophysical electron and tau neutrinos.
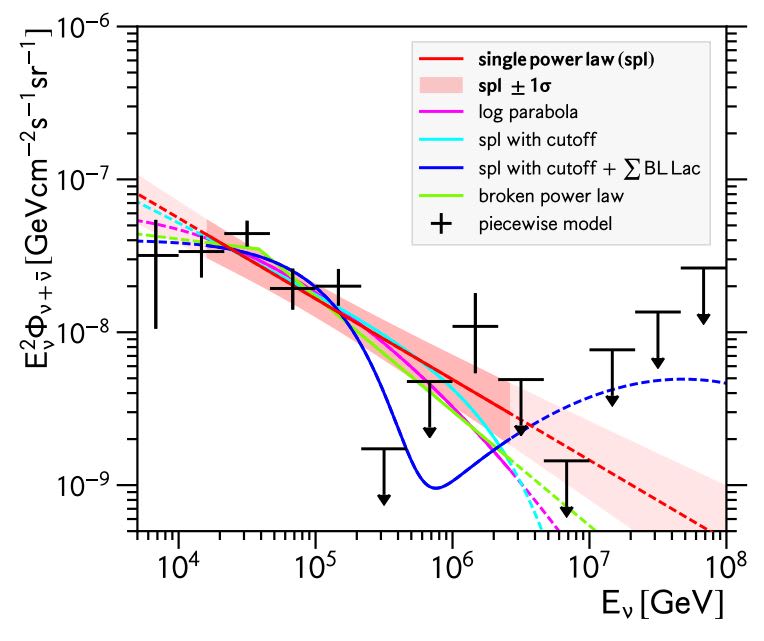
The IceCube Neutrino Observatory detects the presence of neutrinos using 5,160 digital optical modules (DOMs) that are buried in a cubic kilometer of ice at the South Pole. As neutrinos pass through the ice around the DOMs, they sometimes shatter a hydrogen or oxygen nucleus, producing particles that continue to fly through the ice trailed by a cone of Cherenkov light. This light triggers the DOMs as it passes, creating an “event.”
The different flavors of neutrinos tend to produce different event signatures in the detector. A “track” occurs when a muon neutrino interacts in or near the IceCube array, producing a high-energy muon that travels a long distance and leaves an elongated track of signals in its wake. An electron neutrino or tau neutrino that interacts inside the detector and produces electrons or tau particles are observed as “cascades”; they deposit their energy in a small region (about 10 meters) between DOMs, resulting in a nearly spherical event. Measuring cascades also provides insight into the flavor composition of the diffuse neutrino flux, something that scientists have been researching intensely.
For this analysis, IceCube researchers chose to use six years of IceCube cascade data. The analysis builds on methods developed in previous IceCube cascade searches, but the researchers were able to significantly improve it by applying a machine-learning technique to classify background and signal events according to their topology, resulting in a sample with high signal purity.
They found that in the 16 TeV (trillion eV) – 2.6 PeV (quadrillion eV) energy range, the contribution from astrophysical electron and tau neutrinos strongly dominates (roughly 90 percent) over the small contribution from astrophysical muon neutrinos in the cascade samples. Assuming the simplest astrophysical flux model, where the flux varies as a power of neutrino energy (the “single power-law” baseline model), this combined flux of electron and tau neutrinos from the entire sky is in agreement with previous IceCube results that use muon neutrinos and all-neutrino flavors. For the first time, this analysis allowed researchers to characterize the astrophysical electron and tau neutrino flux beyond this baseline model.
Researchers were able to measure the flux more precisely in the Southern Hemisphere than in the Northern Hemisphere, but the two results are consistent. If they had observed a significant difference, it could have provided evidence for galactic and extragalactic origins of this flux.
One unexpected outcome of this analysis was that the obtained results hint at deviations from the baseline model. The flux appears to decrease faster at high energies (~100 TeV) and to increase more slowly at low energies than expected from the baseline model fit. But the researchers point out that more statistics are needed to make a definite statement. If confirmed, this could mean that different neutrino production mechanisms dominate at different neutrino energies. For example, cascades at PeV energies might have different origins than cascades with energies below 100 TeV. In addition, the large measured flux below ~100 TeV may suggest the existence of “hidden” neutrino sources or dense environments, which challenges models of cosmic accelerators that are optically thin to GeV-TeV gamma rays and opens a new avenue in multimessenger astronomy.
Finally, the event sample preselected by this new dedicated cascade analysis will significantly enlarge the existing sample of cascades reported by IceCube to date. As such, it will yield sensitivity to the flavor composition of the astrophysical neutrinos.
info “Characteristics of the diffuse astrophysical electron and tau neutrino flux with six years of IceCube high energy cascade data,” IceCube Collaboration: M. G. Aartsen et al., Physical Review Letters 125 (2020) 12, 121104, journals.aps.org, arxiv.org/abs/2001.09520