On a dark, clear night, you can look up and see the Milky Way galaxy: billions of stars shining in visible light. But we also expect our galaxy to “shine” in neutrinos, elusive particles whose origins are still mysterious. There are cosmic-ray sources within our galaxy, so these sources must also produce neutrinos.
We cannot see neutrinos with our eyes, but the IceCube Neutrino Observatory can detect them. IceCube “sees” with 5,160 optical sensors buried deep in glacial ice at the South Pole.
When neutrinos pass through IceCube, they will sometimes leave signals, known as “events,” primarily as either tracks or cascades. The former occur when a neutrino collides with matter in or near IceCube, resulting in a high-energy muon that travels a long distance, leaving an elongated “track” of signals in its wake. Cascades happen when all or most of the neutrino’s energy is deposited in a small region and results in a nearly spherical event, making it hard to measure the direction from which the parent neutrino came.
Cascades are more difficult to reconstruct than tracks, which are usually used in searches for astrophysical neutrino sources, but they have their own advantages, including providing a better measurement of neutrino energy. By studying cascade events, researchers enhance IceCube’s sensitivity to possible neutrino sources in the southern sky, including the Galactic Center.
In a paper published today in The Astrophysical Journal, the IceCube Collaboration outlined recent results from a source search that used seven years of data from cascade events. While they did not find any statistically significant sources of neutrino emissions, this work is an improvement on the previous source search with cascades.
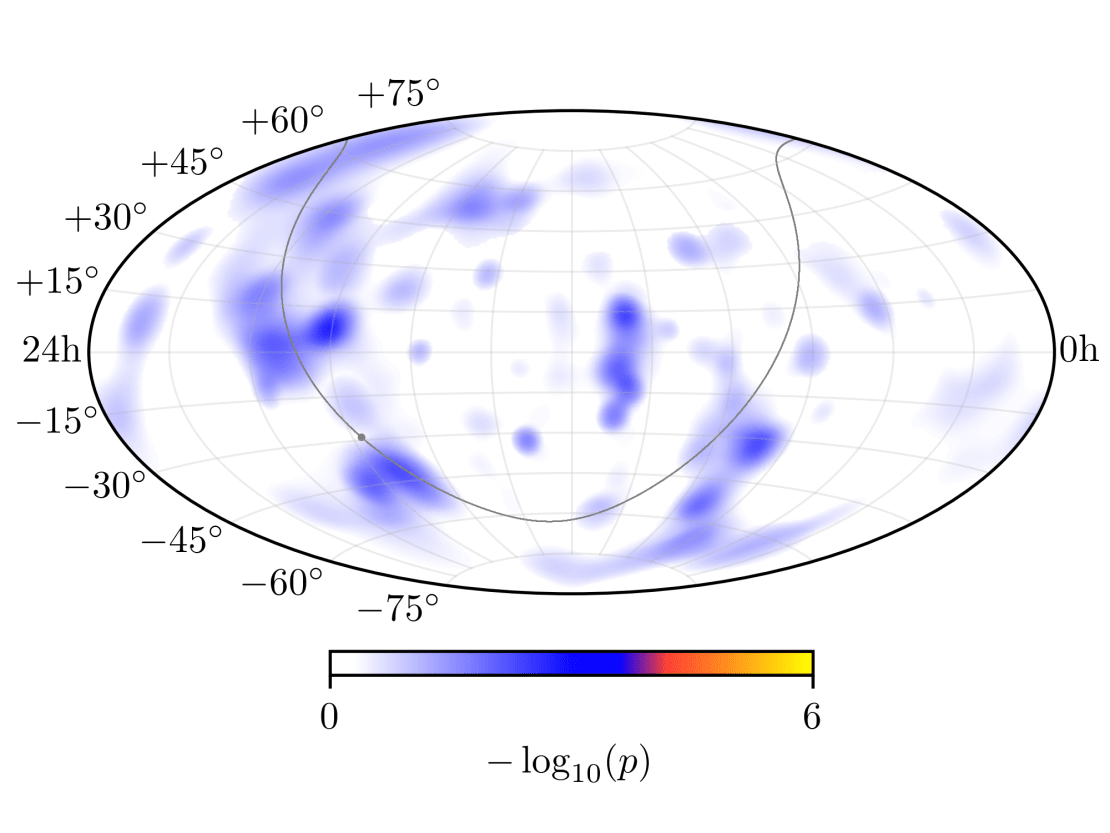
Cascades have the advantage that atmospheric backgrounds are small and relatively uniform throughout the sky. IceCube collaborators previously used two years of cascades in a similar analysis. The current work is an improvement on that analysis in three ways: the use of seven years of data, greatly improved directional reconstruction, and the added emphasis on testing for possible sources within the Milky Way.
To perform their analysis, IceCube scientists first improved the directional reconstruction by using a deep convolutional neural network inspired by recent work in image recognition, rather than the traditional statistical approach. “In principle, the traditional approach should perform better,” says Mike Richman, a postdoctoral researcher at Drexel University and the lead on the analysis, “but in practice, our model of the glacial ice is sufficiently complex that it’s difficult to guarantee that method converges on the optimal result.”
Richman credits fellow IceCube collaborator Mirco Huennefeld of Universität Dortmund for his extensive work on the angular reconstruction used in the analysis. “Mirco has trained a model with an implicit understanding of the detector and the ice, and it’s able to obtain good results without resorting to expensive numerical scans.”
Armed with this improved reconstruction applied to seven years of data, the researchers performed two types of analysis: searches for point sources and searches for broad emission regions in our galaxy. The point source searches included a scan of the whole sky, a scan over 74 preselected potential sources, and a test for sum-total emission from three short lists of interesting supernova remnants. The broad emission regions included gas and dust distributed throughout the Milky Way and the giant “Fermi bubbles” near the center of our galaxy. Many of these tests were the most sensitive performed to date by any experiment.
Ultimately, the researchers did not find evidence for neutrino emission. However, they did acknowledge an interesting trend: As the Milky Way measurements become more sensitive (from using just IceCube tracks, to IceCube tracks and ANTARES events, and now to just IceCube cascades), the result becomes increasingly significant. Furthermore, the galactic neutrino energy spectrum suggested by the cascade data agrees with previous IceCube work with tracks. While not conclusive, this is consistent with emission that is just below the sensitivity of analyses done so far.
This work solidifies the importance of using all neutrino flavors to search for sources—at least with current-generation detectors. Going forward, Richman says they plan to improve the cascade analysis by applying the latest reconstructions to data collected over more time and extending to even lower energies (below 1 TeV). They expect data from the IceCube Upgrade to reduce systematic uncertainties, leading to still better sensitivity.
In the future, the plan is to study additional source types, including ones with time-dependent—and potentially very short-lived—emission. They also plan to combine IceCube tracks and cascades and ultimately to perform a “global” analysis that includes all event types from all available data.
info “Search for sources of astrophysical neutrinos using seven years of IceCube cascade events,” IceCube Collaboration: M. G. Aartsen et al., The Astrophysical Journal 886 (2019) 1, iopscience.iop.org, arxiv.org/abs/1907.06714