Cosmic rays are high-energy particles from outer space; they range in mass from light particles like protons to heavy nuclei like iron. Where exactly they come from is still a mystery, though last year IceCube found evidence that blazars are one source. When a cosmic ray enters Earth’s atmosphere (the “primary” cosmic ray), it produces showers of secondary particles, including electrons, photons, and muons. Some of these secondary particles reach the IceCube Neutrino Observatory, an array of 5,160 optical modules embedded in a cubic kilometer of ice at the South Pole.
The IceCube Collaboration uses data from these cosmic ray air showers to measure the energy and composition of the incoming primary cosmic rays. This is important for scientists’ understanding of cosmic rays because features in the energy spectrum or differences in the relative amounts of light or heavy particles provide clues about what these particles are and where they come from.
In a paper recently published in Physical Review D, the IceCube Collaboration reports on measurements of the all-particle cosmic ray energy spectrum and composition in the PeV to EeV energy range using three years of data from the IceCube Neutrino Observatory.
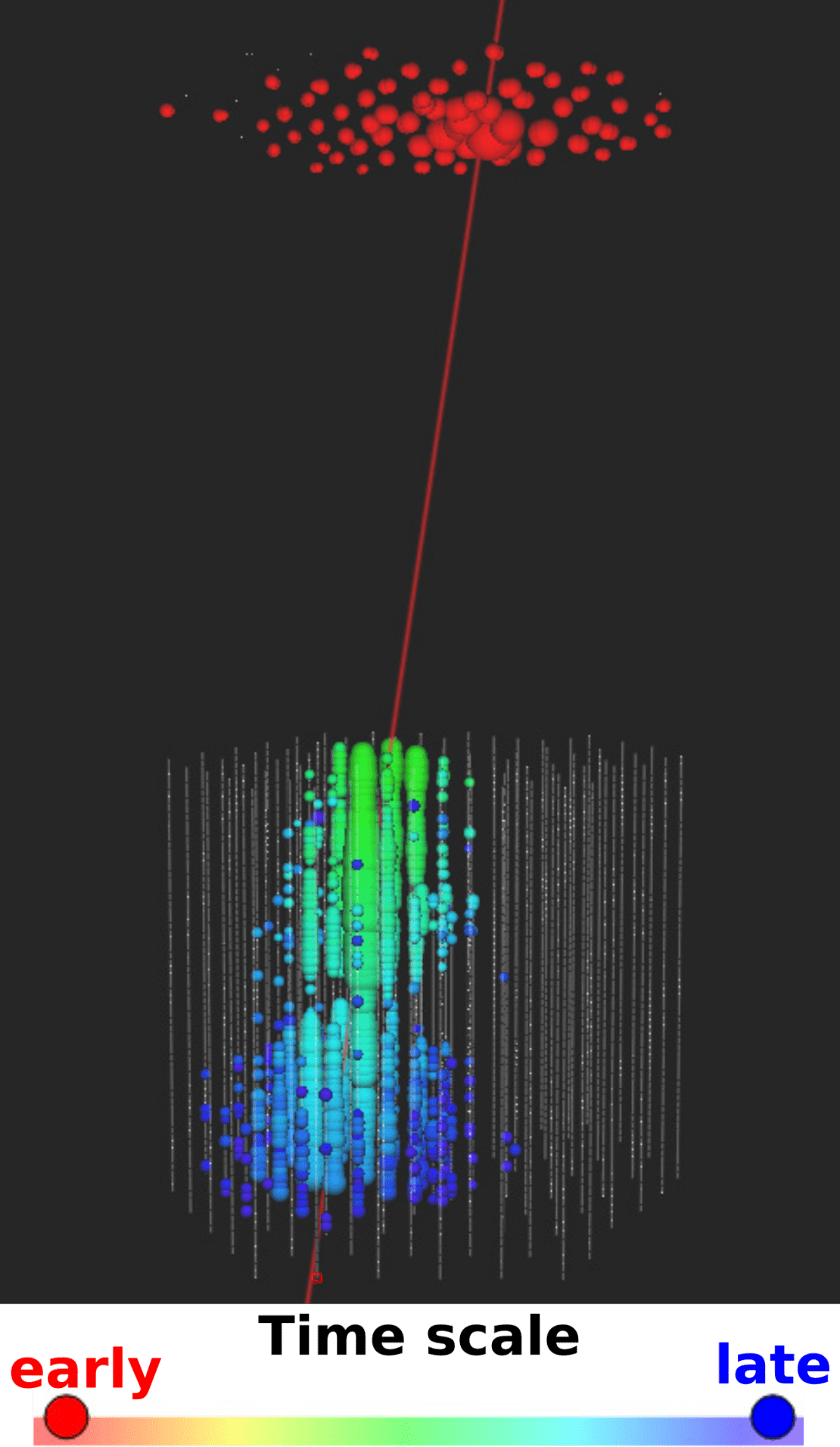
In addition to being a powerful neutrino observatory, IceCube is a unique facility for detecting cosmic rays. This is thanks to components both in the ice and on the ice’s surface. The more than 5,000 digital optical modules (DOMs) on 86 strings make up the in-ice component. The surface component, IceTop, is an array of stations above each string, where each station consists of a pair of tanks that contain two DOMs embedded in frozen water.
Scientists can use the in-ice and surface components to determine the cosmic ray energy spectrum. In this paper, IceCube collaborators used data taken from June 2010 through May 2013 to make measurements in two different ways: an IceTop-alone analysis and a coincident analysis that used the in-ice array in tandem with IceTop.
With IceTop alone, researchers extracted energy spectra using the core position, direction, and size of cosmic ray air showers at the surface. The higher the energy of the primary cosmic ray, the greater the number of secondary particles it produces in the atmosphere, which leads to brighter and more extensive light in IceTop’s tanks. By carefully analyzing the relationship between energy and detected light, researchers can estimate the primary energy of every event detected by IceTop.
Meanwhile, the in-ice detector measures the energy loss of the secondary muons in the deep ice, which is strongly dependent upon the mass of the incident primary cosmic ray. Thus, the IceTop and in-ice detectors were able to measure the high-energy muon component of the secondary air showers in coincidence with the electromagnetic component. This enabled the scientists to measure both the energy spectrum and the energy-dependent mass composition without making assumptions about one to determine the other, as they had to do with the IceTop-alone analysis.
But the coincident analysis had its shortcomings, too. For it to work, the researchers required events that hit both IceTop and the in-ice components of the detector. This was only possible for a small subset of events within the IceTop-alone data, so the coincident analysis was limited in the number of events it could use.
Each of the two approaches had advantages and disadvantages, but the researchers ultimately found that the energy spectra resulting from the IceTop-alone and coincident analyses are in good agreement with each other.
The composition analysis results were a significant improvement over previously published results. The researchers report a higher flux in the iron group at high energies as compared to measurements from other experiments, which could indicate that the iron nuclei are the highest energy “representatives” of a particular population of cosmic rays.
“This analysis provides a composition measurement from a single experiment over a wider range in energy than is accessible by any other single detector to date,” says Karen Andeen of Marquette University, the lead on this paper. “This energy range is particularly interesting because in this regime we expect that there is a transition between cosmic rays produced by galactic sources and cosmic rays produced outside the galaxy, but this transition is not well understood.
“These results represent many years of hard work, and we are very excited to share them with the community.”
info “Cosmic ray spectrum and composition from PeV to EeV using 3 years of data from IceTop and IceCube,” IceCube Collaboration: M. G. Aartsen et al., Physical Review D 100, 082002 (2019), journals.aps.org, arxiv.org/abs/1906.04317