Fast radio bursts (FRBs) are some of the most enigmatic phenomena in the universe. These millisecond-long pulses of radio waves most likely originate outside of our galaxy, but we don’t know much more than that. What causes FRBs has been a stubborn mystery in astrophysics since they were first discovered in 2007. There are many models that attempt to explain them, but the scarcity of FRB sightings and multiwavelength follow-up detections mean that none of the models is strongly favored.
Certain theoretical models suggest that FRBs could be sites of cosmic ray acceleration. We can test this by probing for high-energy neutrinos whose emission is coincident in time with FRBs. These neutrinos—nearly massless particles that can fly through space at close to the speed of light—can be detected with the IceCube Neutrino Observatory, an array of over 5,000 light detectors buried in a cubic kilometer of ice at the South Pole.
When neutrinos pass through the IceCube array, they will sometimes hit an atom of ice and produce a cone of light that triggers the detectors, called an “event.” The IceCube Collaboration recently looked for neutrino events that coincided with 28 nonrepeating FRBs and one repeating FRB. Searching for neutrinos emitted from the same part of the sky as FRBs could provide clues to help test those models that suggest particle acceleration near the FRB source.
Results from this search are outlined in a paper published today in The Astrophysical Journal, “A Search for MeV to TeV Neutrinos from Fast Radio Bursts with IceCube.” In the absence of any significant correlation, they were able to set the world’s best constraints on high-energy neutrino emission from FRBs. In addition, they introduced a wider range of neutrino energies, performing the first search for FRB neutrino signals at megaelectronvolt (MeV) energies.
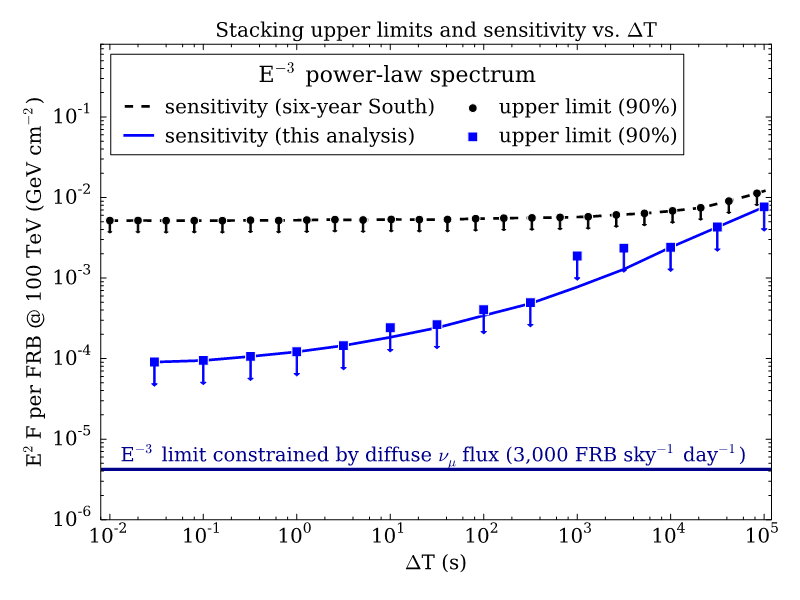
For this analysis, members of the IceCube Collaboration, led by researchers from the Wisconsin IceCube Particle Astrophysics Center at the University of Wisconsin–Madison, performed two searches.
They first built upon a previous search that used six years of IceCube data. In the new search, they looked for spatial and temporal correlations between FRBs and muon neutrino events, which can be identified by the extended, track-like signatures that particles called muons leave in the IceCube detector as a result of a neutrino colliding with an atom in the ice. A key innovation over the prior search, however, is that they used a much wider selection of IceCube events, enabling much better sensitivity.
In the second search, the researchers looked for MeV-energy neutrino signals coincident with FRBs—the first time an FRB neutrino search has ever been carried out at these energy levels (MeV = one million electronvolts). “While IceCube is primarily designed to see neutrinos with energies that are orders of magnitude higher than MeV, it can detect MeV-neutrino signals if it measures a collective rise above the background across many sensors, which is a technique that has been developed for real-time identification of supernovae,” says Ali Kheirandish, a postdoctoral researcher at WIPAC and one of the analyzers of this work. “This provides a unique opportunity to probe for a physical phenomenon emerging at MeV energies.”
No significant association was found between FRBs and neutrinos in either search. However, the researchers were able to set upper limits on the time-integrated neutrino flux from FRBs. These are the most constraining limits to date, including the first upper limits on MeV-neutrino emission from FRBs.
While neither of these analyses boasts a discovery, they are far from precluding the eventual detection of neutrinos from FRBs. The number of detected FRBs is expected to increase rapidly over the next few years, and future searches for neutrinos from FRBs may lead to interesting detections. For now, the results of this paper leave astrophysicists wondering not only about the true nature of FRB progenitors but also about the origins of the astrophysical neutrinos that IceCube detects.
According to WIPAC researcher and UW–Madison professor Justin Vandenbroucke, who helped lead the analysis, “Fast radio bursts and astrophysical neutrinos are both fields of research that are rapidly developing, and establishing a link between them would be a major discovery for each. Although we didn’t find any connection between them in this analysis, we developed new analysis techniques that will be even more powerful as the rate of FRB detections continues its dramatic growth over the next few years.”
info “A Search for MeV to TeV Neutrinos from Fast Radio Bursts with IceCube,” IceCube Collaboration: M. G. Aartsen et al., The Astrophysical Journal 890 (2020) 2, iopscience.iop.org, arxiv.org/abs/1908.09997