Today, the IceCube Collaboration announces a new observation of high-energy neutrinos that originated beyond our solar system. This study, which looked for neutrinos coming from the Northern Hemisphere, confirms their cosmic origin as well as the presence of extragalactic neutrinos and the intensity of the neutrino rate. The first evidence for astrophysical neutrinos was announced by the collaboration in November 2013. The results published now in Physical Review Letters are the first independent confirmation of this discovery.
“Looking for muon neutrinos reaching the detector through the Earth is the way IceCube was supposed to do neutrino astronomy, and with this paper, it delivered,” says Francis Halzen, principal investigator of IceCube and the Hilldale and Gregory Breit Distinguished Professor of Physics at the University of Wisconsin–Madison. “It is not quite CMS and ATLAS, but this is as close to an independent confirmation as one can get with a single instrument.”
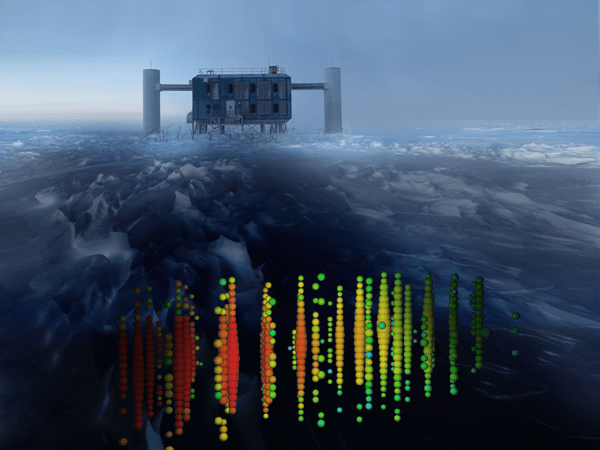
Neutrinos are subatomic particles that travel throughout the universe almost undisturbed by matter, pointing directly to the sources where they were created. And for the highest energy neutrinos, those sources are expected to be the most extreme environments in the universe: powerful cosmic generators, such as black holes or massive exploding starts, that are able to accelerate cosmic rays to energies over a million times the energies achieved by record-breaking human-made accelerators, such as the LHC at CERN.
“Cosmic neutrinos are the key to yet unexplored parts of our universe and might be able to finally reveal the origins of the highest energy cosmic rays, including the rare ‘Oh-My-God’ particles,” says collaboration spokesperson Olga Botner, of Uppsala University. “The discovery of astrophysical neutrinos hints at the dawn of a new era in astronomy.”
Neutrinos are never directly observed, but IceCube is able to see the by-products of a neutrino interaction with the Antarctic ice. This cubic-kilometer detector records a hundred thousand neutrinos every year, most of them produced by the interaction of cosmic rays with the Earth’s atmosphere. Billions of atmospheric muons created in the same interactions also leave traces in IceCube. And from all of these, researchers are looking for only a few dozen astrophysical neutrinos, which will expand our current understanding of the universe.
The search presented today by the IceCube Collaboration uses an old strategy for a neutrino telescope: it looks at the universe through the Earth, using our planet to filter the large background of atmospheric muons. More than 35,000 neutrinos were found in data recorded between May 2010 and May 2012. At the highest energy, above 100 TeV, the measured rate cannot be explained by neutrinos produced in the Earth’s atmosphere, indicating the astrophysical nature of high-energy neutrinos. The analysis presented in this paper suggests that more than half of the 21 neutrinos above 100 TeV are of cosmic origin.
This independent observation, with a significance of 3.7 sigma and in good agreement with previous results by the IceCube Collaboration, also confirms the high rate of astrophysical neutrinos. Even though scientists are still counting them by the handful, IceCube results are close to the maximum rates based on potential cosmic ray sources. The intensity of this flux shows that cosmic ray sources are also efficient generators of neutrinos. And, therefore, these tiny particles are further endorsed as the perfect tools to explore the extreme universe.
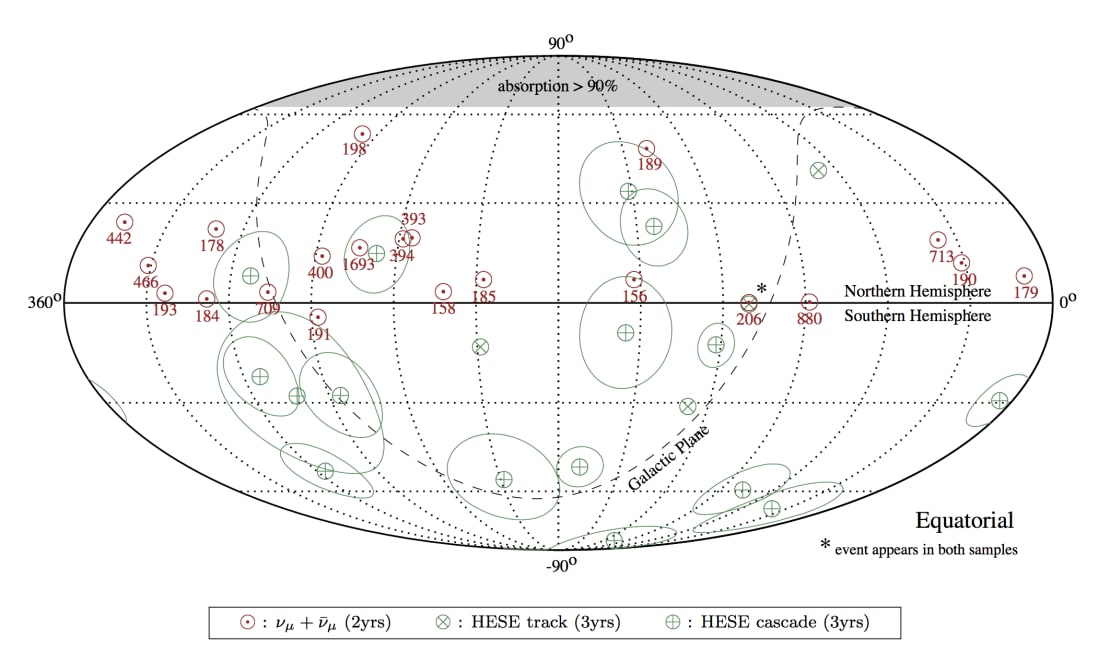
The observed high-energy neutrinos are a brand-new neutrino sample, with only one event in common with the first results announced in 2013, which searched for high-energy neutrinos that had interacted with the ice inside IceCube during the same data-taking period. The current search looked for muon neutrinos only. These neutrinos produce a muon when they interact with the ice and have a characteristic signature in IceCube, called a track, that makes them easy to identify. The same shape is expected for an atmospheric muon, but by looking only at the Northern Hemisphere, researchers know that a detected muon could have only been produced by a neutrino interaction.
These neutrino-induced tracks have a very good pointing resolution, in which they can locate their sources within less than a degree. However, IceCube’s studies have not yet found a significant number of neutrinos coming from any single source. The neutrino flux measured by IceCube in the Northern Hemisphere has the same intensity as the astrophysical flux in the Southern Hemisphere. This adds support to a large population of extragalactic sources, since otherwise sources in the Milky Way would dominate the flux around the galactic plane.
In addition, this new high-energy neutrino sample, when combined with previous IceCube measurements, allows the most accurate measurements to date of the energy spectrum and neutrino-type composition of the extraterrestrial neutrino flux. Those results are published in an accompanying paper in The Astrophysical Journal.
IceCube, run by the international IceCube Collaboration and headquartered at the Wisconsin IceCube Particle Astrophysics Center (WIPAC) at UW–Madison, is a gigaton particle detector located near the Amundsen-Scott South Pole Station, one of the scientific facilities in Antarctica managed by NSF. It is buried beneath the surface, extending to a depth of about 2,500 meters. A surface array, IceTop, and a denser inner subdetector, DeepCore, significantly enhance the capabilities of the observatory, making it a multipurpose facility.
The IceCube Neutrino Observatory was built under an NSF Major Research Equipment and Facilities Construction grant, with assistance from partner funding agencies around the world. The NSF’s Division of Polar Programs and Physics Division continue to support the project with a Maintenance and Operations grant, along with international support from participating institutions and their funding agencies. UW–Madison is the lead institution, and the international collaboration includes 300 physicists and engineers from the U.S., Germany, Sweden, Belgium, Switzerland, Japan, Canada, New Zealand, Australia, U.K., Korea and Denmark.
+ info “Evidence for Astrophysical Muon Neutrinos from the Northern Sky with IceCube,” IceCube Collaboration: M.G. Aartsen et al. Physical Review Letters 115, 081102 (2015).
Contacts:
Francis Halzen, PI IceCube
Hilldale and Gregory Breit Distinguished Professor of Physics
University of Wisconsin–Madison
francis.halzen@icecube.wisc.edu
Olga Botner, IceCube Spokesperson
Professor of Physics
University of Uppsala
olga.botner@physics.uu.se
Note: A multimedia gallery is available at icecube.wisc.edu/gallery/press
The IceCube Collaboration has also released data related to this paper.
Read here about the first evidence for astrophysical neutrinos (Science, November 2013)
“A combined maximum-likelihood analysis of the high-energy astrophysical neutrino flux measured with IceCube,” IceCube Collaboration: M. G. Aartsen et al. The Astrophysical Journal 98 (2015). iopscience.iop.org arxiv.org