Neutrinos are ghostly, nearly massless particles that can travel extraordinarily large distances unimpeded. Because of this, neutrinos act as “messengers,” harboring information about their sources. Although most detected neutrinos originate from the sun or Earth’s atmosphere, there exist highly energetic astrophysical neutrinos that originate from the farthest reaches of outer space.
In 2013, the first evidence of high-energy astrophysical neutrinos originating from cosmic accelerators was seen by the IceCube Neutrino Observatory, which houses a cubic-kilometer-sized neutrino telescope buried deep within the Antarctic ice. These cosmic messengers provide insight into their sources, which are some of the most powerful cosmic objects in the universe. Since these neutrinos have to travel billions of light-years in vacuum, they can also act as barometers of quantum gravity—the unification of gravity, forces, and space-time.
Today, the IceCube Collaboration presents the results of its search for the effect of quantum gravity on the flavors of astrophysical neutrinos. Although no evidence was found for quantum gravity effects, the result presented is the first to reach the expected signal region of quantum gravity using neutrino flavor interferometry. This study was published today as a letter in the journal Nature Physics.
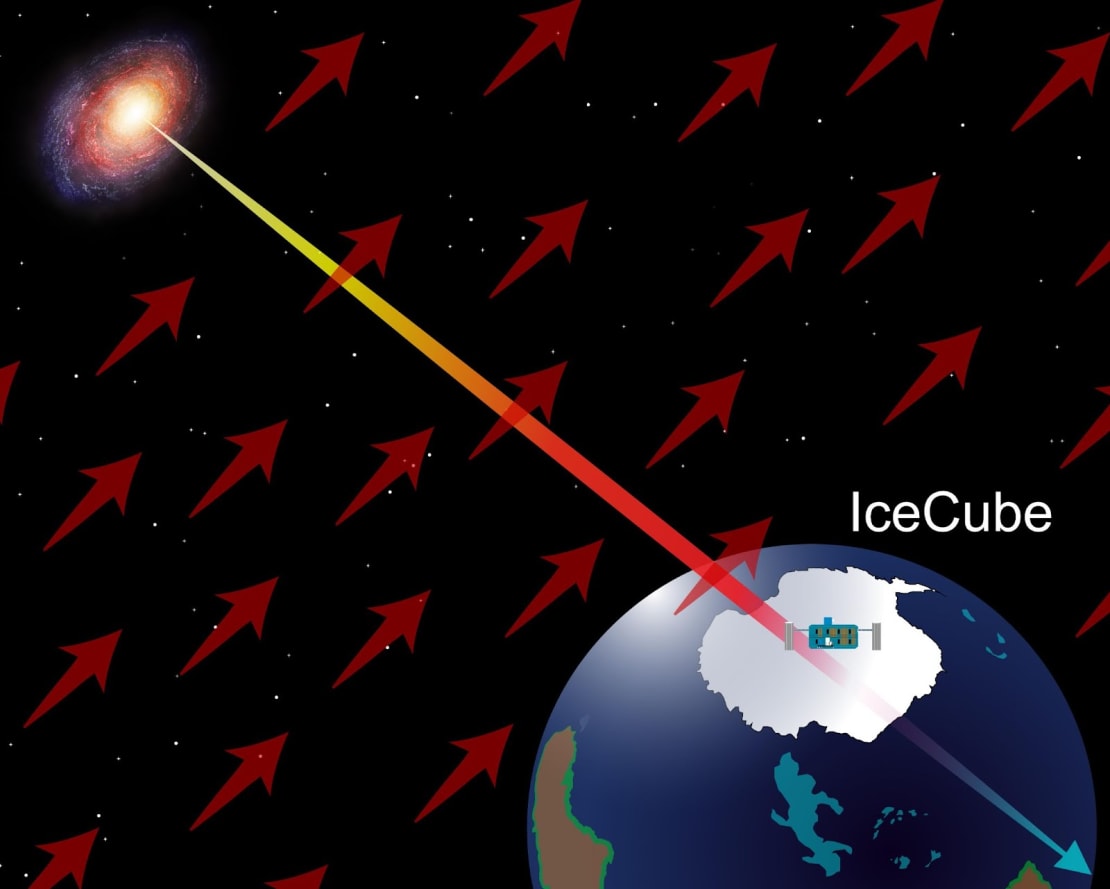
“The nature of space and time, later joined by Albert Einstein as space-time, has been a question that has puzzled thinkers since the Greek philosophers. Using interferometric measurements, they could probe the vacuum’s content,” said Carlos Argüelles, an assistant professor of physics at Harvard University and a lead on the analysis. “Similarly, more than a hundred years later, we can use neutrino interference to search for the signatures of new, unexpected content in vacuum.”
Neutrinos come in three different flavors: electron, muon, and tau. As astrophysical neutrinos propagate from their distant sources, they can oscillate or convert to another flavor due to quantum interference. Because these neutrinos are sensitive to tiny defects in the vacuum, these perturbations can be used to hunt for space-time structures.
To conduct the search, researchers used 7.5 years of IceCube’s high-energy starting events (HESE) that include some of the highest astrophysical neutrinos ever observed by IceCube. The data was then used to measure the neutrino flavor composition on Earth.
“For the simulation, we generated the astrophysical neutrino flavor model to include the quantum gravity effect,” said Teppei Katori, an associate professor of physics at King’s College London and a lead on the analysis. “This simulation used the Standard-Model Extension, a theoretical framework that describes all possible new space-time effects in a consistent way.”
“Neutrino interference changes the fraction of the flavors observed in IceCube. The flavor ratio is one of the most powerful probes for new physics since it has a well-established expectation in the Standard Model background,” explained Argüelles. “This is what you want to have when you are searching for new physics: we need a good prediction of our Standard expectation so that we can distinguish that from deviations produced by new physics.”
The measured neutrino flavors were then compared against the expected neutrino flavors resulting from quantum interference during their propagation. A Bayesian framework was used to compare the simulation against the data.
Overall, the study found no evidence of anomalous flavor conversion in the IceCube data that would hint at quantum gravity effects. Nonetheless, researchers were able to place the most stringent limits of any known technologies on the dimension-six operators that parameterize the space-time defects. With the completion of IceCube-Gen2, a next-generation neutrino telescope 10 times larger than the current IceCube array, on the horizon, Katori is confident that the analysis will improve with enhanced data acquisition.
“We can also improve the analysis by developing a better algorithm to measure astrophysical neutrino flavors,” said Katori. “The combination of these improvements will push this analysis toward the discovery of quantum gravity.”
+ info “Search for Quantum Gravity Using Astrophysical Neutrino Flavour with IceCube,” IceCube Collaboration: R. Abbasi et al., Nature Physics (2022), DOI:10.1038/s41567-022-01762-1