Ever since the first detection of gravitational waves by LIGO and Virgo, the IceCube Neutrino Observatory has been searching for neutrino counterparts to gravitational waves. Detecting both gravitational waves and neutrinos from the same source would be a groundbreaking step in multimessenger astronomy, the field that uses multiple kinds of cosmic messengers to see the universe in different ways. But it hasn’t happened yet.
Until recently, high-energy neutrino observatories like IceCube have mostly searched for neutrino counterparts in the 1012 to 1015 electronvolt range (TeV to PeV). But some models predict that gravitational wave sources could produce neutrinos at lower energies—energies that have not been extensively explored—on the scale of 109 electronvolts (GeV).
Now, thanks to a newly developed GeV neutrino selection method, IceCube researchers can access that lower energy range. Today, the IceCube Collaboration submitted a paper to Physical Review D that outlines the results of a search for GeV neutrinos from possible gravitational wave events detected by LIGO and Virgo. They did not find evidence of GeV neutrino counterparts, but the researchers look forward to continuing the search as more gravitational wave candidates are found.
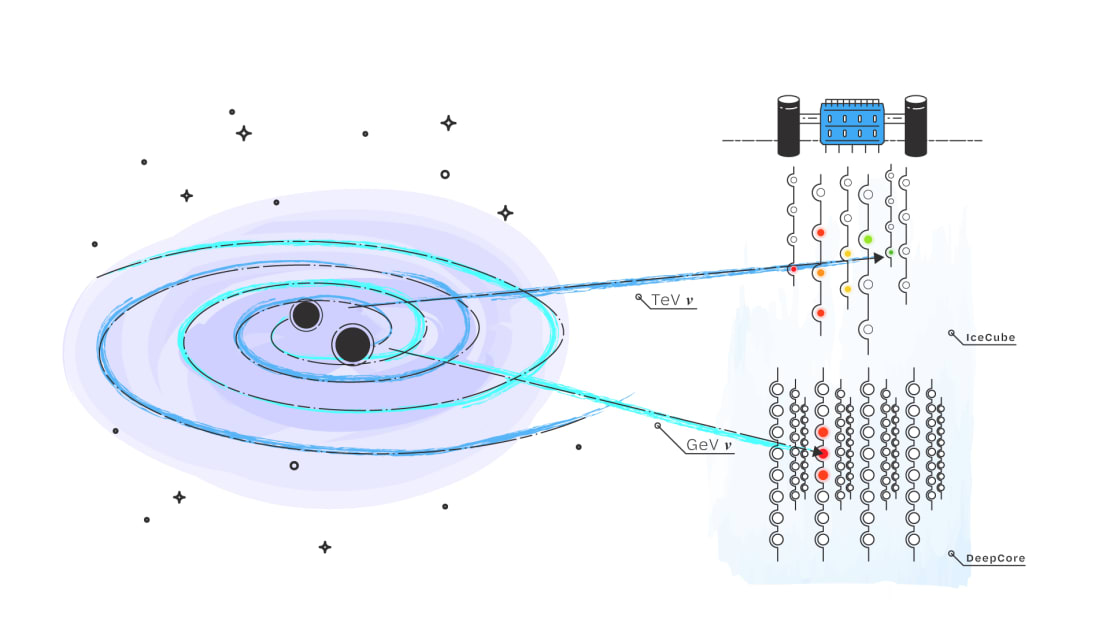
That first gravitational wave detected in 2015 came from a binary black hole merger: the meeting of two black holes that had closely orbited each other. This is one kind of a gravitational wave-producing phenomenon known as a “compact binary merger”; other types include binary neutron star mergers, neutron star–black hole mergers, and “mass-gap” mergers.
In this analysis, IceCube collaborators used the new GeV selection method on IceCube data recorded during all the compact binary merger candidates that were detected by the LIGO and Virgo interferometers during their first three observing runs. For most of the 70 merger candidates, the researchers looked for an unusual increase in the number of events detected by IceCube in a 1,000-second time window surrounding the time of the merger. While they found no significant excess, they were able to place upper limits on the expected GeV neutrino flux. They also looked at all merger candidates stacked together to see if there may have been sources emitting GeV neutrinos that were too faint to see individually, but they found no sign of such a population.
The researchers treated a few mergers differently. When there might have been a significant amount of matter surrounding a source, they searched for a “prompt” signal by only looking in the three seconds after the merger time. Again, they found nothing unusual, and thus derived an upper limit.
Though this search didn’t reveal any GeV neutrinos coincident with gravitational waves, the paper shows the expanded energy range in which IceCube is providing useful constraints in the hunt for neutrinos from our universe.
“Even though we have not found any significant neutrino signal yet from merger candidates, we have validated our follow-up strategy in the GeV range and are getting ready for the future observing runs of the LIGO, Virgo, and now KAGRA (Kamioka Gravitational Wave Detector) interferometers,” says Gwenhaël de Wasseige, a Marie Curie individual fellow at APC Laboratory in Paris and a lead on this analysis. “With many more gravitational wave candidate events, we will have lots of opportunities to search for a GeV neutrino signal.”
+ info “Probing neutrino emission at GeV energies from compact binary mergers with the IceCube Neutrino Observatory,” IceCube Collaboration: R. Abbasi et al., Submitted to the Physical Review D, arxiv.org/abs/2105.13160