Neutrino oscillations were discovered by Super-Kamiokande in 1998, proving that neutrinos had nonzero mass and that new physics was hiding behind these tiny, only weakly interacting particles.
Since then, several experiments have measured neutrino oscillations––including IceCube––mainly as the appearance or disappearance of electron- and muon-type neutrinos. However, a fuller understanding of this quantum morphing requires precise measurements of the appearance or disappearance of tau neutrinos.
The IceCube Collaboration has just performed its first measurement of tau neutrino appearance in oscillations of atmospheric muon neutrinos, which excluded the absence of tau neutrino oscillations at a significance of 3.2σ, confirming previous observations by OPERA and Super-Kamiokande. More importantly, this result is also the leading measurement of the tau neutrino “normalization,” the ratio of the detected number of tau neutrinos arising from oscillations to the predicted number from the standard neutrino oscillation picture. The measured tau “normalization” is consistent with the standard picture but suggests that future IceCube measurements of this quantity could reveal new physics. These results have just been submitted to the journal Physical Review D.
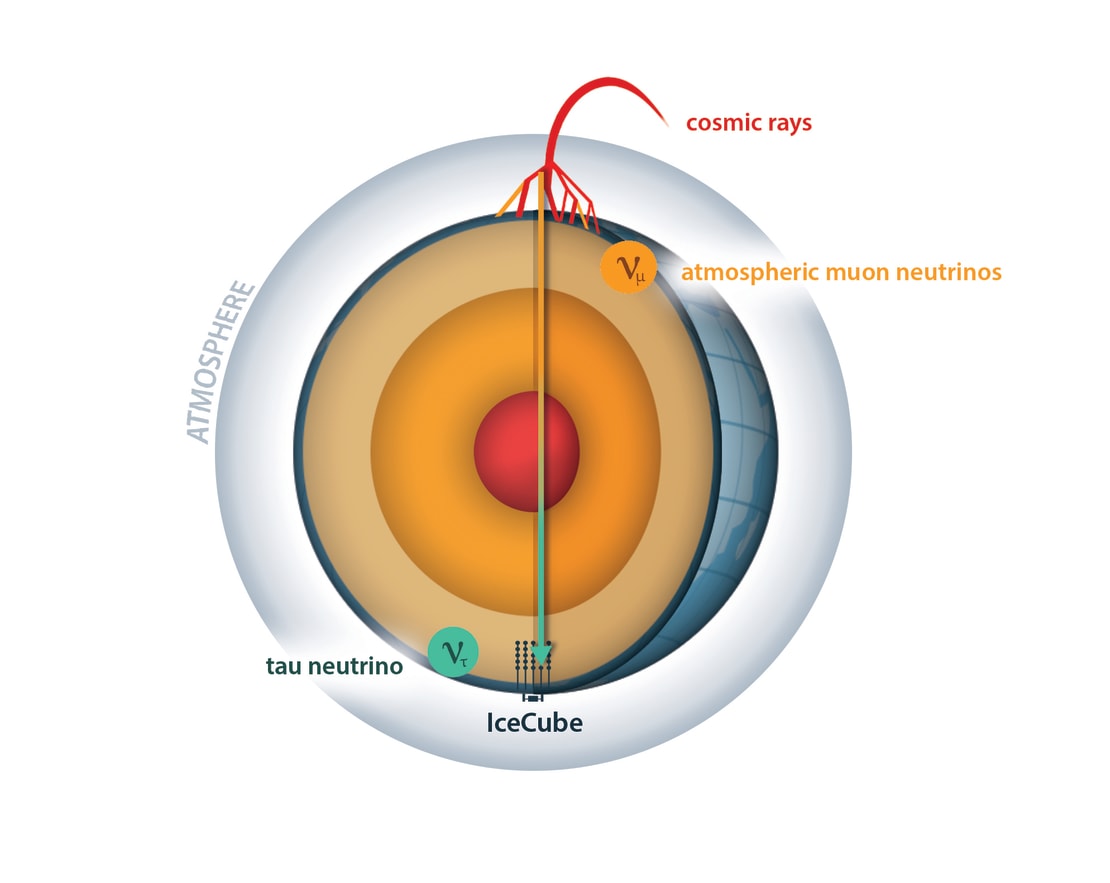
We know of the existence of three neutrino types or “flavors,” namely electron, muon, and tau. Theories that predict a fourth type of neutrino or other sorts of nonstandard anomalies in the neutrino sector could provide answers to important open questions in physics, such as the origin of the matter-antimatter asymmetry in the universe and the small masses of neutrinos.
Precise measurements of the oscillation patterns of the three neutrinos are required to confirm or rule out the possibility of new physics. Many experiments have measured electron and muon neutrino appearance and disappearance with great precision, and efforts continue to achieve sensitivities that could reveal new physics. In addition, given that producing a beam of tau neutrinos is still out of reach for particle accelerators and that the number of detected astrophysical tau neutrinos will be small for the foreseeable future, the measurements of oscillations for the third neutrino have concentrated on the appearance of tau neutrinos in oscillations of electron and muon neutrinos.
The OPERA experiment, using a muon neutrino beam from CERN in Switzerland to Gran Sasso in Italy, was the first to observe tau neutrino appearance, with five tau neutrinos detected five between 2010 and 2015. In 2017, Super-Kamiokande confirmed this detection using atmospheric neutrinos.
The IceCube measurement of tau neutrino appearance using three years of atmospheric data confirms these detections and points the way toward an even more precise measurement of this fundamental parameter. Researchers used atmospheric neutrinos created in the interaction of cosmic rays with the Earth’s atmosphere. These neutrinos contain negligible numbers of tau neutrinos. Neutrinos are detected indirectly once they interact in the DeepCore subarray, located in the bottom center of IceCube, deep in the Antarctic ice sheet. Atmospheric neutrinos reaching IceCube have traveled distances ranging from 20 to 13,000 km, depending on whether they were created in cosmic-ray interactions in the Southern or the Northern Hemispheres. For those traveling the longer distances, a high probability for converting to the tau flavor is expected at energies around 25 GeV.
Tau neutrino appearance is suppressed in charged current interactions due to the large tau lepton mass, which is much greater than both electron and muon masses. This means that below 3.5 GeV the interaction cannot take place, and above this threshold it is roughly a factor of 10 times smaller than for the other two flavors. In IceCube’s high-statistics neutrino sample, several hundred tau neutrinos are expected to interact in DeepCore every year.
The IceCube measurement looked for tau neutrino appearance at energies between 5.6 and 56 GeV. At these energies, the exclusive, event-by-event identification of a tau neutrino is not possible in IceCube since a tau lepton resulting from a charged current interaction will decay after only 1 mm of flight, creating a cascade signature that is indistinguishable from that created by a charged current interaction of electron neutrinos and neutral current neutrino interactions of any flavor.
The tau neutrino appearance is measured as variations in the number of track-like and cascade-like events produced by the oscillations of atmospheric muon neutrinos as a function of energy and incoming direction––the latter correlates with the distance traveled by the incoming neutrinos. These two parameters, energy and distance traveled, are used to determine the neutrino oscillation rate.
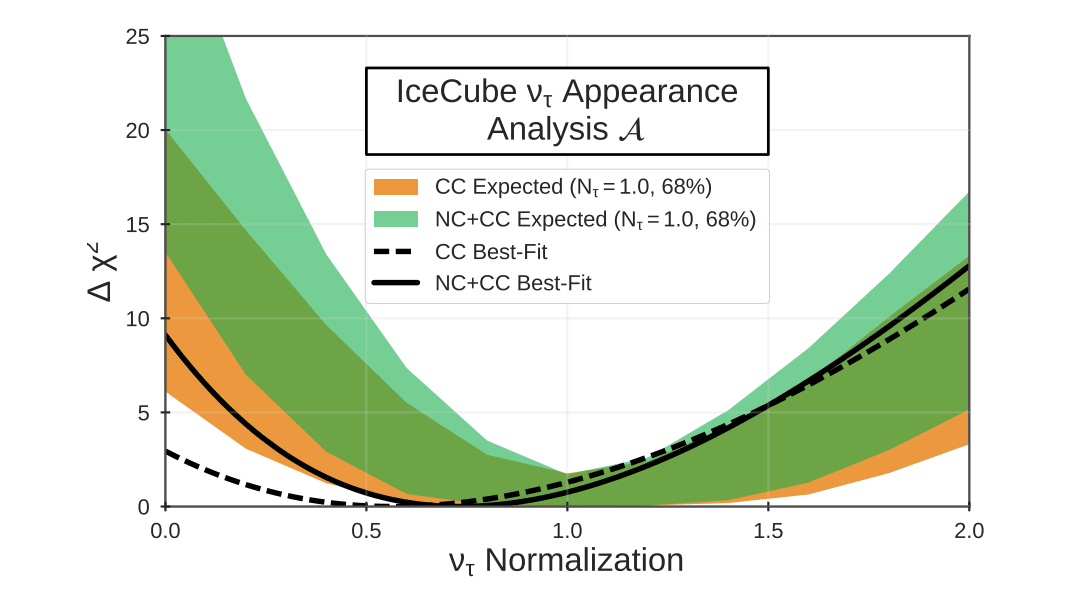
The measurements allowed excluding the absence of oscillations into tau neutrinos at a 3.2 sigma level. Scientists also looked for deviations from the standard oscillation model by measuring the precise rate of tau neutrino appearance in comparison with the standard oscillation with three neutrino flavors. The appearance rate ratio was measured to be 0.73 (+0.34, -0.24), compatible with the standard oscillation paradigm within the uncertainty of the measurement.
A larger data sample of more than 10 years of IceCube data will strongly improve this measurement. More importantly, the enhanced reconstruction of low-energy neutrinos with the current detector and with the planned IceCube Upgrade––expected to be deployed by early 2023––will allow a measurement of the tau neutrino normalization with a precision of better than 10% with a single year of operation. If the central value of the tau neutrino normalization remains at 0.73, a measurement with 10% precision would be strongly suggestive of new physics.
info “Measurement of Atmospheric Tau Neutrino Appearance with IceCube DeepCore,” IceCube Collaboration: M.G. Aartsen et al., Physical Review D 99, 032007 (2019), journals.aps.org, arxiv.org/abs/1901.05366.