Science relies on the premise that nature’s laws are the same for anyone running an experiment. This may seem obvious to someone sitting on a couch at home, but for many of the objects we study—huge or tiny—that are fleeting through space and matter, it might not be the case.
Scientists call this property Lorentz symmetry—that the laws of physics stay the same as long as observers move at constant speed, no matter the direction, with respect to each other—and many theories, including general relativity, rely on it. A well-known consequence of this symmetry is that the speed of light remains constant at 300 million meters per second.
Several models trying to unify under one single theory both quantum theory and gravitation predict Lorentz violation at extremely, but significant, small scales. And, as one might guess, bizarre neutrinos could be the particles to reveal which theory is correct. However, for now, we don’t have an answer. A new measurement of the IceCube Collaboration has put Lorentz symmetry to the test and found—yet again—that neutrinos behave as expected. The results, published in Nature Physics, are the most stringent limits to date in the neutrino sector on the existence of a Lorentz violating field.
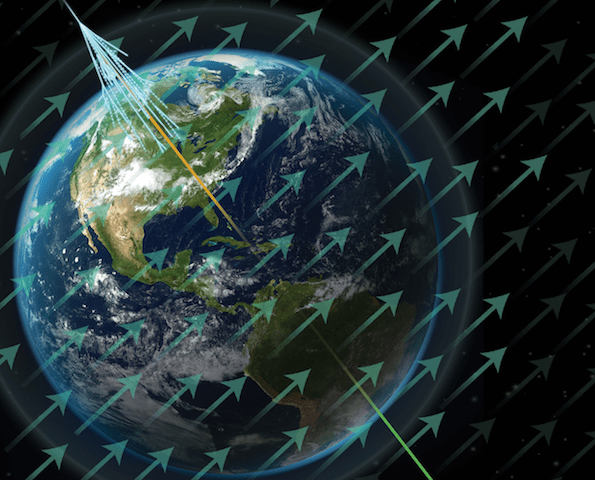
Since they were first postulated, neutrinos have been considered as tantalizing signatures of yet to be discovered new physics. The observation of neutrino oscillations––one of the most interesting quantum phenomena––answered positively to whether these ghostly particles had mass while at the same time generating more puzzles to be solved.
In a universe where the Lorentz symmetry can be breached, such as a universe where gravity can also be explained with quantum theories, the morphing of neutrinos from one type, or flavor, to another would deviate from standard neutrino oscillations. The effects of the interaction of neutrinos with a Lorentz violating field, or the aftermath of a small distortion of space-time, would be enhanced by the quantum interference of the different flavors and result in significant changes on the oscillation pattern.
“Neutrino oscillations are a natural interferometer,” explains Teppei Katori, an assistant professor at Queen Mary University of London and one of the main analyzers of this work. And IceCube neutrinos are the ones with the highest energies ever the detected, produced by cosmic-ray protons colliding with atoms in the Earth’s atmosphere or coming directly from distant and powerful cosmic accelerators. “Neutrino oscillations observed with IceCube act as the biggest interferometer in the world to look for the tiniest effects in physics, such as a space-time deficit,” adds Katori.
It was a legendary interferometer, the so-called Michelson and Morley experiment, that using a beam of light proved that space was not full of ether. A better theory to understand the nature of space was proposed by Einstein, and since then we understand space and time to be in a four-dimensional continuum.
“We would love to be the direct descendants of Michelson and Morley, using interferometry to show that a beautiful field theory, in this case Einstein’s theory, is right, but not sufficient—that there is new physics out there that underlies our present model,” says Janet Conrad, a professor at MIT who also participated in this study.
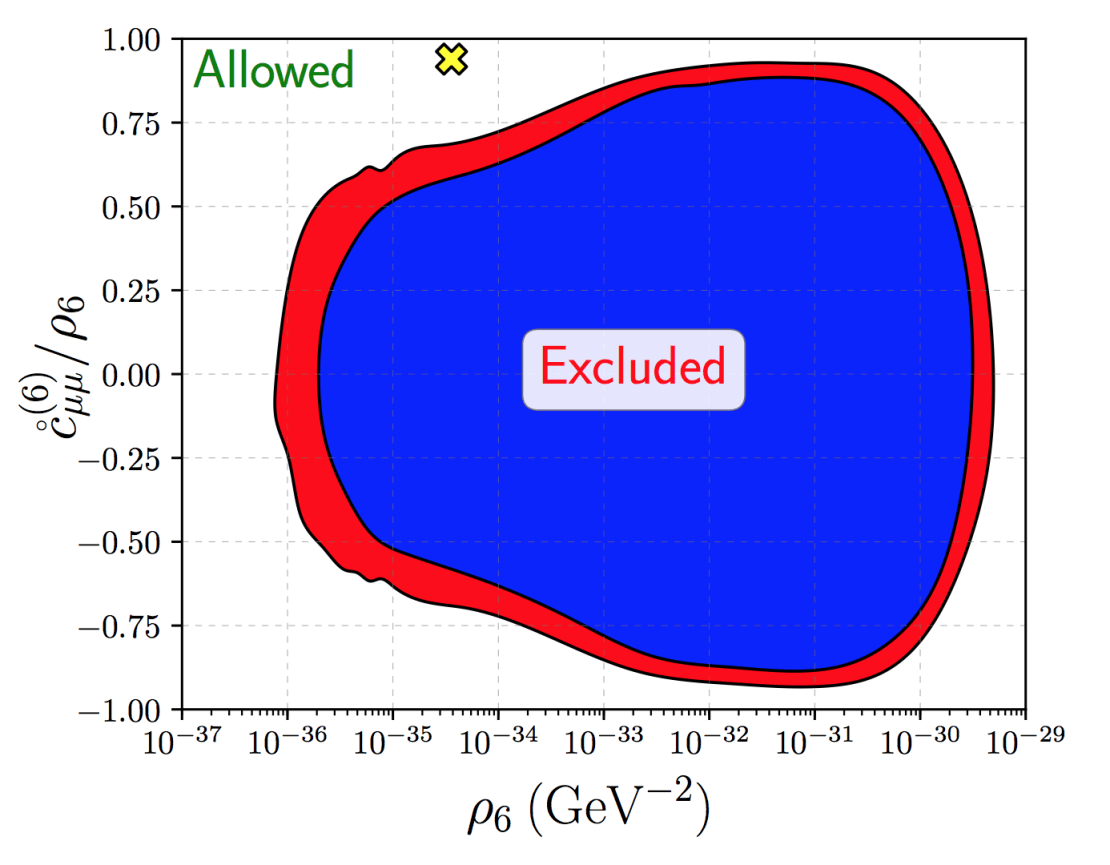
IceCube researchers have looked for effects of an isotropic Lorentz violating field using two years of throughgoing atmospheric neutrinos with energies around and above one TeV. The results have not found variations in the typical neutrino oscillation patterns that could be pointing to new physics.
“We have looked for missing muon neutrinos on the highest energy atmospheric events observed by IceCube and have found no significant deficit,” says Carlos Argüelles, a postdoctoral researcher at MIT and also a main analyzer of this work. “We’re getting closer to the Planck scale: an unexplored terrain where general relativity and quantum mechanics are both important. Exciting times lie ahead as we continue looking for strange flavor changes at higher and higher energies.”
This nonobservation allowed setting the strongest limits to date on Lorentz violating fields in the neutrino sector. When looking at results from other types of experiments, these limits are also among the best in the world, showing the potential of neutrino experiments to study fundamental space-time properties.
“IceCube’s access to the high-energy regime, along with its high statistics, makes it a powerful instrument to study physics beyond the standard model. This enabled us to probe for small distortions induced by Lorentz invariance violation in the atmospheric neutrino flux that are not accessible to other detectors,” says Ali Kheirandish, a postdoctoral researcher at UW–Madison and also an analyzer of this work.
Improvements to these measurements are already in the works. The addition of astrophysical neutrinos will increase sensitivity to higher energies, where many searches for new physics could reach the precision to prove or rule out some theories.
The IceCube analysis focused on an isotropic Lorentz violating field since its impact on neutrino oscillation signatures may be expected to be up to 1,000 larger than the impact from a directional field. However, as sensitivity increases, IceCube will also test directional fields, which are considered a smoking gun signature of Lorentz violation. Future extensions of IceCube as well as water-based neutrino detectors will provide larger samples of astrophysical neutrinos and will boost tests of the most fundamental symmetries in nature.
+ info “Neutrino interferometry for high-precision tests of Lorentz symmetry with IceCube,” IceCube Collaboration: M.G. Aartsen et al., Nature Physics (2018), DOI:10.1038/s41567-018-0172-2, arXiv