A denser and smaller array of sensors at the bottom of the IceCube Neutrino Observatory, the DeepCore detector, enables the detection of neutrinos produced by the interaction of cosmic rays with the atmosphere down to energies of only a few GeVs. On their way to IceCube, many of the neutrinos produced in the Northern Hemisphere will morph into other neutrinos due to a well-known quantum effect: neutrino oscillations.
In 2013, IceCube reported its first measurement of the neutrino oscillation parameters. This was the first time that neutrino oscillations were measured with precision at energies between 20 and 100 GeV. The results were compatible with those from devoted neutrino experiments, but now the model was tested at higher energies, although uncertainties were still larger. A year later, the collaboration presented a second analysis with three years of data that improved the precision by a factor of ten. This week, the IceCube Collaboration presents a new measurement of the oscillation parameters that for the first time is competitive with the best measurements to date. These results have just been submitted to Physical Review Letters.
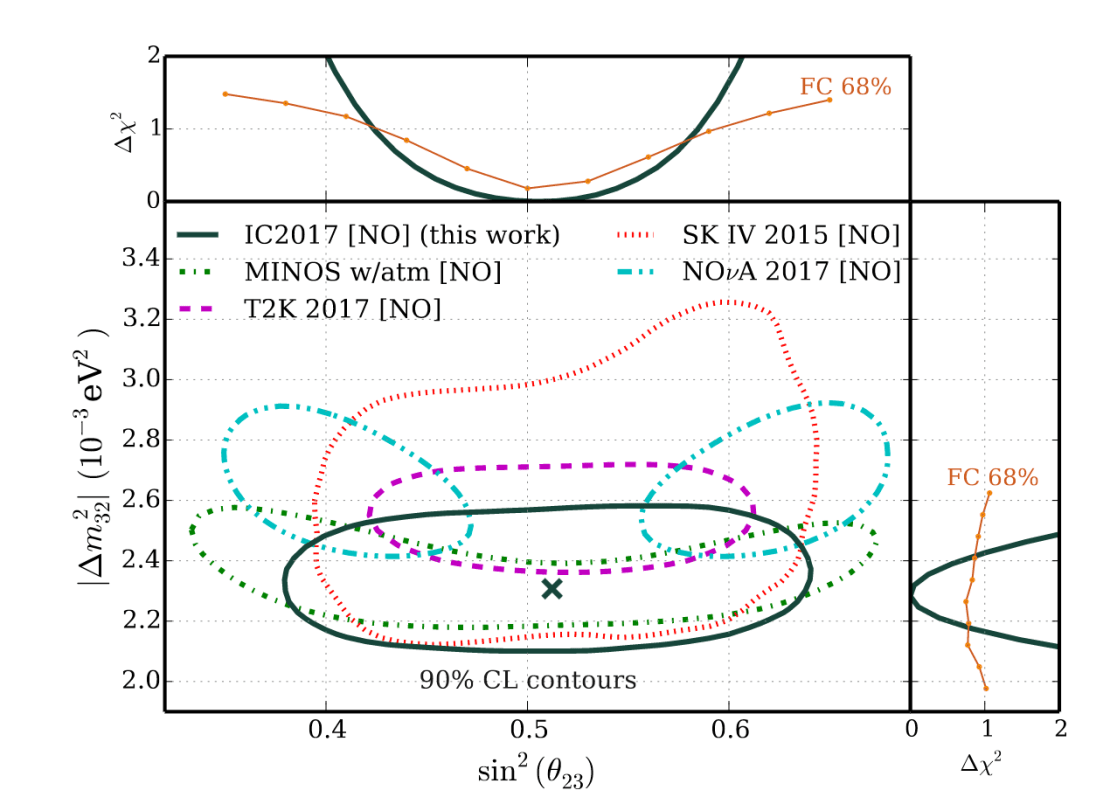
Long-baseline experiments, such as T2K or NOvA, observe much lower energy events. Understanding neutrino oscillations at higher energies tests systematic uncertainties but also places constraints on different new physics models in the neutrino sector.
The current measurement has improved the selection of neutrino events by a factor ten. The IceCube sensors immediately surrounding DeepCore are used as a veto against muons produced in the same atmospheric cosmic ray interactions, keeping only events that start inside the DeepCore instrumented volume.
“The event reconstruction is a significant improvement of this analysis,” explains João Pedro Athayde Marcondes de André, an IceCube researcher at Michigan State University (MSU) and a coleader of this analysis. “We now take into account the properties of the ice to reconstruct all types of events, even those with a substantial energy deposition at the beginning of the event, where the interaction of the incoming neutrino with the Antarctic ice takes place,” adds A. M. de André.
“IceCube is the first experiment using atmospheric neutrinos to measure the oscillation parameters with a similar precision to long-baseline experiments,” says Joshua Hignight, also an IceCube researcher at MSU and a coleader of this work. “But we measure them in a different energy range and with different baselines,” states Hignight.
The best fit oscillation parameters point to a maximal mixing scenario, in agreement with results from the T2K experiment and in tension with measurements from the NOvA experiment. In the maximal mixing scenario, one of the neutrino quantum states is a precise equal mix of two different flavor neutrinos. Although this could be just a coincidence, it could also be a hint to new physics.
+ info “Measurement of Atmospheric Neutrino Oscillations at 6-56 GeV with IceCube DeepCore,” IceCube Collaboration: M. G. Aartsen et al., Physical Review Letters 120, 071801 (2018). journals.aps.org, arxiv.org/abs/1707.07081
+ Read the Synopsis on APS Physics: Putting Neutrino Oscillations on Ice