Since the first detection of neutrinos with PeV energies, IceCube researchers have performed several follow-up studies to investigate the nature of the astrophysical neutrino flux. These analyses have revealed, for instance, that this flux extends down to energies around 25 TeV and that it displays different event topologies.
The IceCube Collaboration is now revisiting these results in a combined analysis accepted for publication in The Astrophysical Journal. The analysis is based on the results of six individual studies and uses up to three observables—energy, zenith angle and event topology—to derive improved constraints on the energy spectrum and the composition of neutrino flavors (νe , νμ , ντ) of the astrophysical neutrino flux.
The current study shows that the energy spectrum of the astrophysical neutrino flux is well described by a power law with a best-fit spectral index of -2.50 ± 0.09, for energies between 25 TeV and 2.8 PeV. A continuous power-law spectrum with index -2, which is a popular benchmark model, is excluded with a significance of 3.8 sigma.
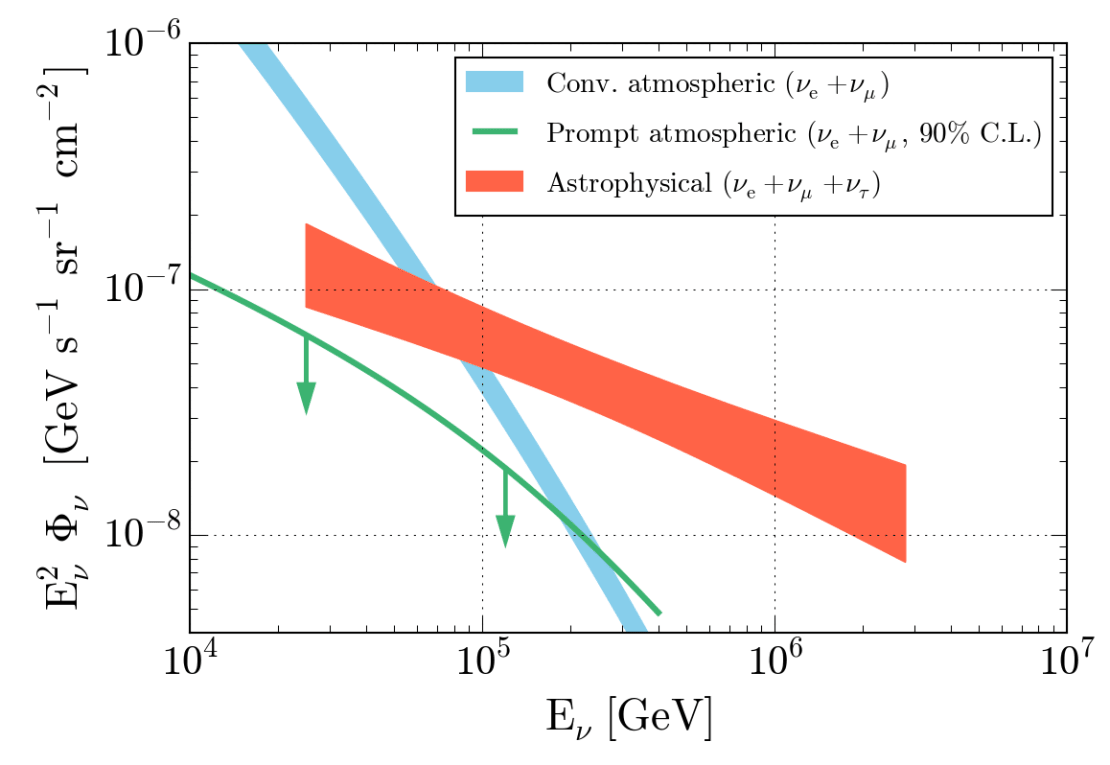
The combined analysis benefits from an increased size of the event sample, which is also more diverse—including track-like as well as shower-like events. Tracks are produced by most interactions of muon neutrinos, in which long-range muons are produced. On the other hand, interactions of electron and tau neutrinos, as well as some muon neutrinos, give rise to shower-like events.
“With this study, we are able to present the first comprehensive characterization of the astrophysical neutrino flux at IceCube,” explains Lars Mohrmann, an IceCube researcher at DESY in Zeuthen and corresponding author of the paper.
The flavor composition of the astrophysical neutrino flux brings us information about the production mechanism and the properties of the neutrino sources. In many scenarios, neutrinos are produced in the decay of pions, which create one electron neutrino per every two muon neutrinos and no tau neutrinos (νe : νμ : ντ =1:2:0). Because neutrinos switch flavors during their long journey through the universe, the 3-flavor composition at Earth is expected to be approximately even (≈1:1:1). The constraints on the flavor composition derived with this study show that the data are compatible with this scenario as well as with the sole production of muon neutrinos (0:1:0).
In another mechanism, astrophysical neutrinos are produced in the decay of neutrons whereby only electron neutrinos are produced (1:0:0). However, this scenario is excluded with a significance of 3.6 sigma.
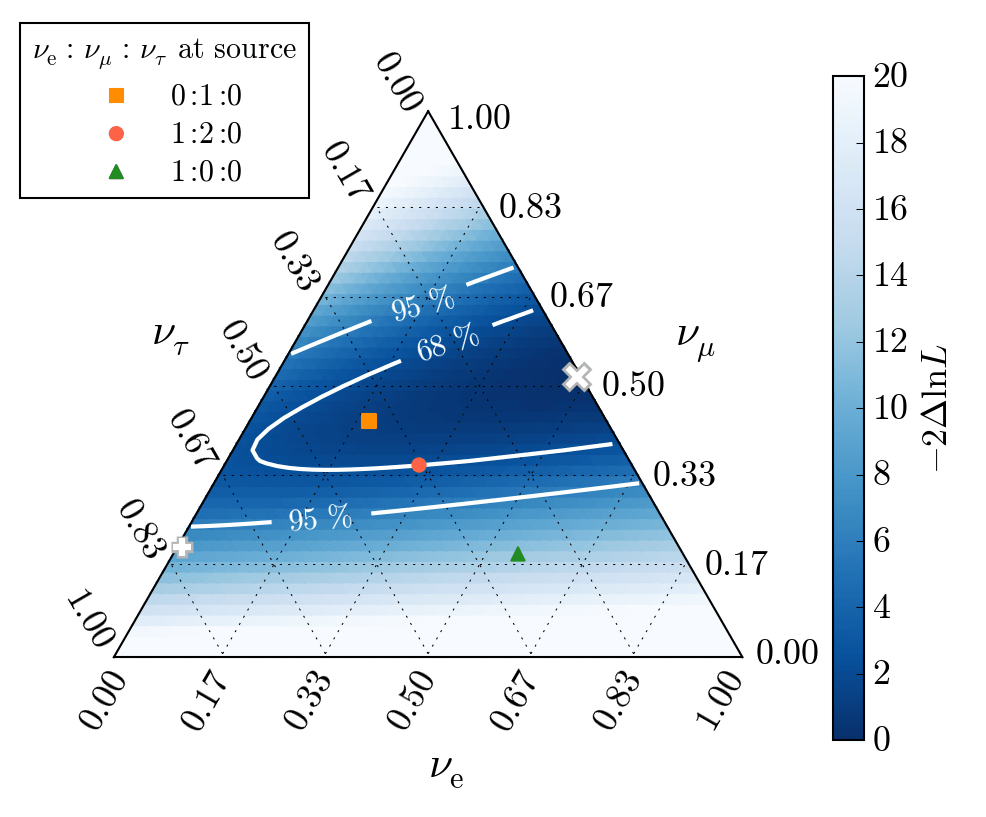
“These results illustrate that we can learn something about the sources of the astrophysical neutrinos, even though we have not identified them yet,” continues Mohrmann. “It will be important to continue investigating the energy spectrum and the flavor composition with more data in the future.”
+ Info: “A combined maximum-likelihood analysis of the high-energy astrophysical neutrino flux measured with IceCube,” IceCube Collaboration: M. G. Aartsen et al. The Astrophysical Journal 809 (2015) 98, iopscience.iop.org