Astrophysical neutrinos are cosmic particles with unique properties. They travel through matter, radiation, and magnetic fields without disruption, bringing us information about their sources in the far and extreme universe. Since their discovery, back in 2013, IceCube researchers are learning to decode what their direction, energy, and flavor can tell us about the most powerful cosmic generators, those that can accelerate cosmic rays to energies above one million times the energy of the Large Hadron Collider (LHC).
Physicists expect that the flavor ratio of astrophysical neutrinos at Earth reflects a nearly even distribution among electron, muon, and tau flavors (1:1:1) . In a new measurement of the flavor ratio of astrophysical neutrinos, submitted today to Physical Review Letters, the IceCube Collaboration has found good agreement with the standard source model. The collaboration also sets limits on nonstandard flavor compositions, which could be a signature for new physics in the neutrino sector, such as neutrino decay or sterile neutrinos.
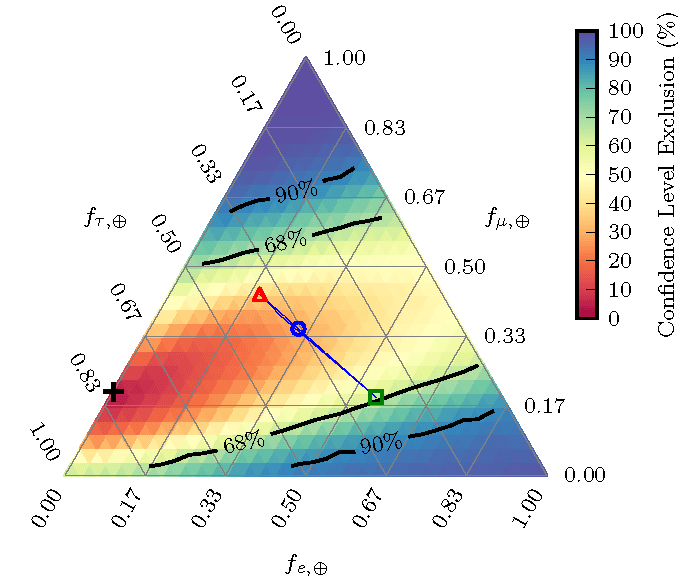
The flavor composition of astrophysical neutrinos allows us to explore the properties of the source environment, such as matter and radiation density or strength of magnetic fields. In the most common source scenario, astrophysical neutrinos will be produced with a ratio of one electron neutrino per every two muon neutrinos (1:2:0). But depending on the source model, this ratio could move from an electron-neutrino-only scenario (1:0:0) to a muon-neutrino-only scenario (0:1:0). No significant production of tau neutrinos is present at the source.
However, the flavor composition of astrophysical neutrinos measured by ground-based detectors such as IceCube will be different due to neutrino oscillations averaged over astronomical distances. In the first scenario, an equal proportion of electron, muon and tau neutrinos is expected at Earth (1:1:1), while this ratio is (0.6:1.3:1.1) for the electron-neutrino-only scenario and (1.6:0.6:0.8) for the muon-neutrino-only scenario. Variations beyond this range would be a signature for new physics in the neutrino sector, such as neutrino decay or Lorentz violation.
The study uses data from May 2010 to May 2013, which had already been used to discover astrophysical neutrinos above 100 TeV. This time the event selection is modified to include shower-like events down to 35 TeV, while the energy cut for track-like events remains as in previous analyses. Lowering the energy cut for shower-like events is possible since atmospheric muons and neutrinos look more track-like at lower energies.
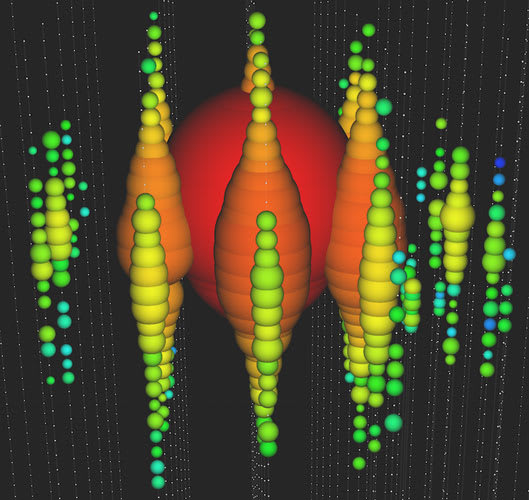
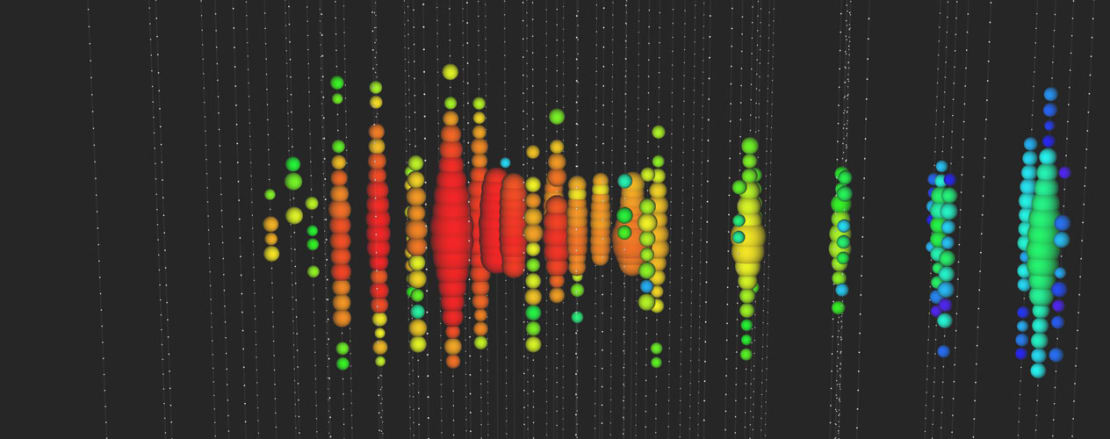
Muons and muon neutrinos will mostly be detected as track-like events, showing the path of the muon—either atmospheric or induced by the neutrino—traveling through IceCube. This is not the case of electron and tau neutrinos, which will be usually detected as shower-like events. Therefore, astrophysical neutrinos are expected to be mostly shower-like in IceCube.
“Though we cannot yet discern between various source models, this measurement illustrates the potential of IceCube in the very near future to give us unique information about the astrophysical environments producing high-energy neutrinos and perform new searches for physics beyond the Standard Model,” notes corresponding author Gary Binder, an IceCube researcher at LBNL and the University of California, Berkeley.
IceCube researchers have also used this data to test some nonstandard compositions, such as an electron-neutrino-only at Earth scenario, or a muon-neutrino-only at Earth scenario. Both have been excluded, at the 2.3 and 3.3 sigma level, respectively.
+ Info “Flavor Ratio of Astrophysical Neutrinos above 35 TeV in IceCube,” IceCube Collaboration: M.G. Aartsen et al. Physical Review Letters 114 (2015) 17, arxiv.org/abs/1502.03376 journals.aps.org