A search for neutrino interactions inside IceCube brought evidence of an extraterrestrial neutrino flux. Now the IceCube Collaboration has expanded the search, lowering the range of deposited energy down to 1 TeV. The goal was a better understanding of the different contributions to the neutrino flux in IceCube and hopefully to measure the charmed-meson component for the first time.
The results of this study, submitted today to Physical Review D, have again proven neutrinos from charmed-meson decays to be elusive. The good news is the results set a more stringent upper limit on their contribution, only 1.52 times the theoretical benchmark prediction used in previous IceCube analyses. IceCube researchers have also derived new constraints on the diffuse astrophysical neutrino spectrum,
$$\Phi_\nu = 2.06_{-0.3}^{+0.4}\times 10^{-18}(E_\nu/10^5GeV)^{-2.46\pm0.12}GeV^{-1}cm^{-2}sr^{-1}s^{-1}$$
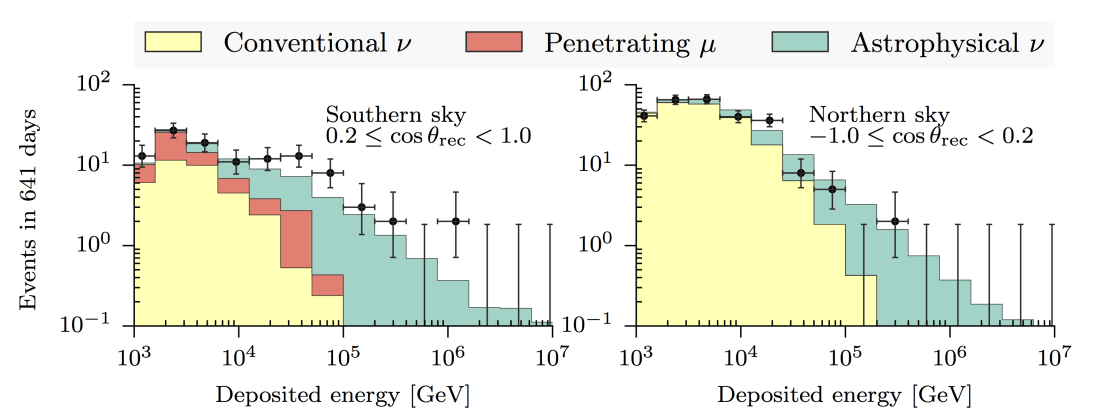
High-energy neutrinos may be produced either by the interaction of cosmic rays in the Earth’s atmosphere, the so-called atmospheric neutrinos, or in the vicinity of distant astrophysical accelerators like black holes and neutrons stars, the so-called astrophysical neutrinos.
Whether atmospheric or astrophysical, neutrinos come in different flavors, but their rates and their energy and direction distributions can help us distinguish the different contributions to the overall flux and learn more about their origins and production mechanisms.
Atmospheric neutrinos are mainly muon neutrinos produced in 2-body decays of charged pions and kaons. The electron neutrino component is primarily due to 3-body decays of charged and neutral kaons. And there’s still a third contribution from the decays of heavy, short-lived mesons containing charm quarks, which create approximately the same amount of muon and electron neutrinos. However, this third prompt contribution has never been conclusively observed.
Astrophysical neutrinos, which may have been created by diffusive shock acceleration in the vicinity of cosmic ray sources, will arrive to Earth with an equal ratio for the three flavors (muon, electron and tau neutrinos) and with an energy spectrum expected to follow that of the progenitor protons (~E-2). Thus, their flux will exceed that of atmospheric neutrinos at very high energies.
The current search was looking for neutrino-induced interactions starting in the IceCube detector with energies between 1 TeV and beyond a few PeV, exploring for the first time the region between 10 and 100 TeV, where neutrinos from charmed-meson decays in the atmosphere should be observable. There were 388 events found in IceCube data from 2010-2012, which should include contributions from conventional and prompt atmospheric neutrinos, and extraterrestrial neutrinos, along with a small but nearly irreducible background of penetrating atmospheric muons that go undetected before depositing a large fraction of their energy in the glacial ice in a single, catastrophic loss.
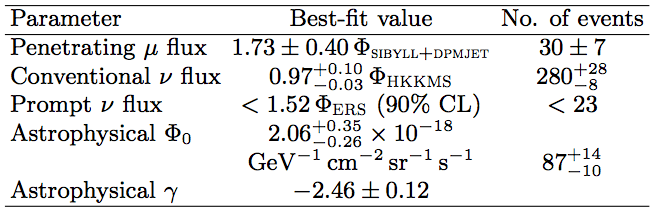
The best fit of each of these contributions to the observed neutrino flux in IceCube shows a good agreement with previous results, except in the region around 30 TeV in the southern sky (see figure and table above) where an extra component might be required to account for the observed events.
“Isolating neutrino interactions is more difficult at these lower energies, but we are seeing interesting things. Some, like the observation of the atmospheric neutrino flux at the observed level, were expected and are a nice confirmation of our understanding of the IceCube detector,” explains Jakob van Santen, a graduate student at WIPAC at the University of Wisconsin–Madison. “Others, like the excess over our simple model of the astrophysical neutrino flux in the southern sky around 30 TeV, a region where we expect our vetoes to remove nearly all atmospheric muons and neutrinos, were a surprise. While the deviation isn’t statistically significant, this may be a hint that our simplest model for the astrophysical neutrino flux is incomplete. To find out more, we’ll need more data.”
The spectral index for astrophysical neutrinos is larger than 2.2, which may be a hint for a galactic neutrino component of this flux. At higher energies, though, neutrinos from extragalactic sources would be the dominant ones. However, the best fit to this index hardens to 2.25 if the energy threshold is raised to 60 TeV, again an indication that we need more data to understand the neutrino flux with energies around 30 TeV in IceCube.
Info “Atmospheric and Astrophysical Neutrinos above 1 TeV Interacting in IceCube,” IceCube Collaboration: M.G. Aartsen et al. Physical Review D91 (2015) 2, journals.aps.org arXiv.org:1410.1749