The first evidence of the massive amount of energy produced by supernovas in a neutrino burst came in 1989 when both the Kamiokande and the IMB detected the resulting neutrinos.
In 1989 the Kamiokande team confirmed Ray Davis’s 1968 results, that the flux of neutrinos from the Sun was indeed much lower than expected, approximately only about a third. At the same time a number of other things were happening. The Frejus detector, located near the center of the Frejus tunnel in France, and the NUSEX experiments, two much smaller installations which used iron instead of water, reported no deficit in muon-neutrino interactions. In addition, the LEP accelerator in Switzerland and the SLC at the SLAC in Stanford both came to the conclusion that only three light neutrino species exist: the electron, the muon, and the tau through the study of the Z boson lifetime.
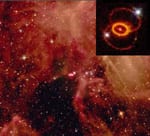
The shorter the lifetime of a particle, the more undetermined its mass is, this mass distribution has a width and this width increases as the number of possibilities for the particle to decay does. The Z boson, whose mean lifetime is around 10-23 seconds, can decay in a pairs particle, an anti-particle such as a neutrino, and an anti-neutrino. The larger the number of neutrino families, the larger the width of the Z mass distribution is.
But experimental particle physicists take a lot of convincing, and there was still the possibility that the solar-neutrino problem arose not from the neutrinos but from the solar models themselves. This is because the neutrino flux measured by the Davis and the Kamiokande experiments was dominated by high-energy neutrinos from a small side reaction involving the decay of boron-8. The rate of this reaction depends critically on the core temperature of the Sun, so a small error in this temperature could explain the low neutrino fluxes seen in both experiments. We therefore had to confirm that all solar neutrinos were suppressed, not just those at high energies.
This required two new experiments called SAGE and GALLEX, which followed the basic idea of Davis’ experiment except that they used gallium instead of chlorine as the target atom. Due to the more complex chemistry involved, these experiments were more difficult to perform, but in the early 1990s we eventually got the answer: the low-energy neutrinos were missing too. The problem did not lie with the solar models – it was something else.
–physicsweb.org