Neutrinos are chargeless, weakly interacting particles that are able to travel undeflected through the cosmos. The IceCube Neutrino Observatory at the South Pole searches for the sources of these astrophysical neutrinos in order to understand the origin of high-energy particles called cosmic rays and, therefore, how the universe works.
IceCube has already shown that neutrinos can exist up to about 10 PeV in energy, but both experimental and theoretical evidence suggests extremely high-energy (EHE) neutrinos should reach higher energies. One component, called cosmogenic neutrinos, are expected to be produced when the highest energy cosmic rays interact with the cosmic microwave background. These EHE neutrinos would have an astounding one joule of energy per particle, or higher.
By understanding the properties of cosmogenic neutrinos, such as their quantity and distribution in energy, scientists are hoping to solve the 100-year-old mystery of the origin of ultra-high-energy cosmic rays (UHECRs), with energies exceeding 1 EeV. In a study submitted to Physical Review Letters, the IceCube Collaboration presents a search for EHE neutrinos using 12.6 years of IceCube data. The nondetection of neutrinos with energies well above 10 PeV improves the upper limit on the allowed EHE neutrino flux by a factor of two, the most stringent limit to date. The collaborators also used the neutrino data to probe UHECRs directly. This analysis is the first result using neutrino data to disfavor the hypothesis that UHECRs are composed only of protons.
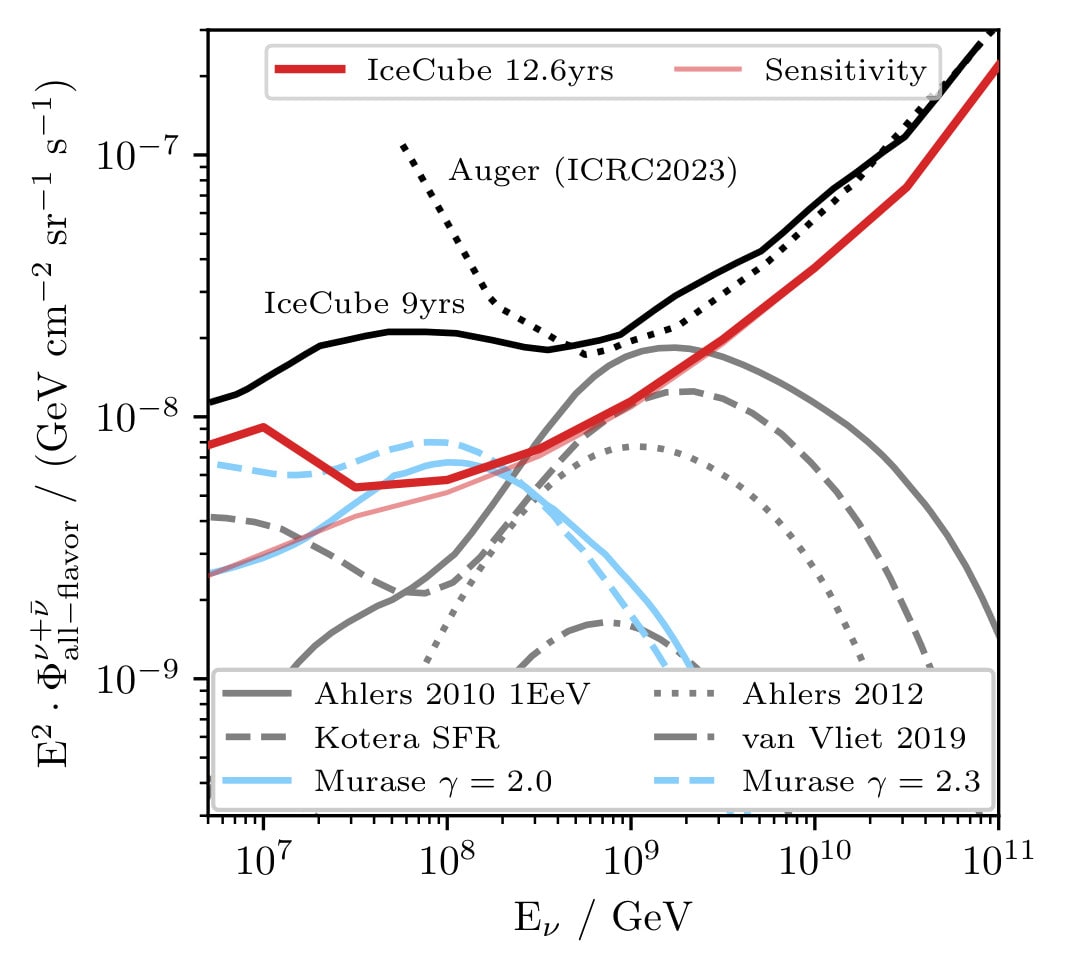
In the search for EHE neutrinos, researchers looked for neutrino “events” where neutrinos deposited a huge amount of light inside the detector. However, because most high-energy neutrinos are absorbed by the Earth, the focus of the study shifted to neutrinos arriving sideways at (horizontal) or above (downgoing) IceCube. Focusing on horizontal events in particular also allowed the researchers to eliminate most of the overwhelming background of atmospheric muons caused by cosmic-ray interactions above IceCube in the atmosphere.
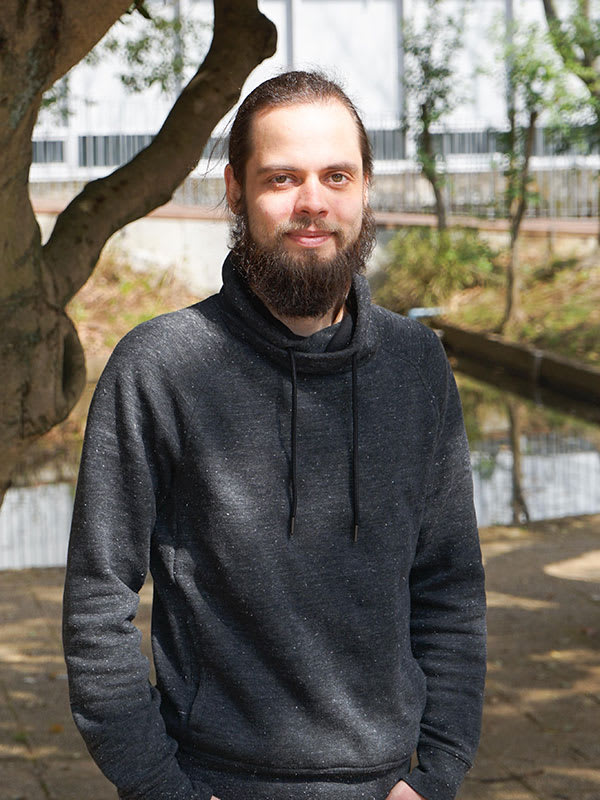
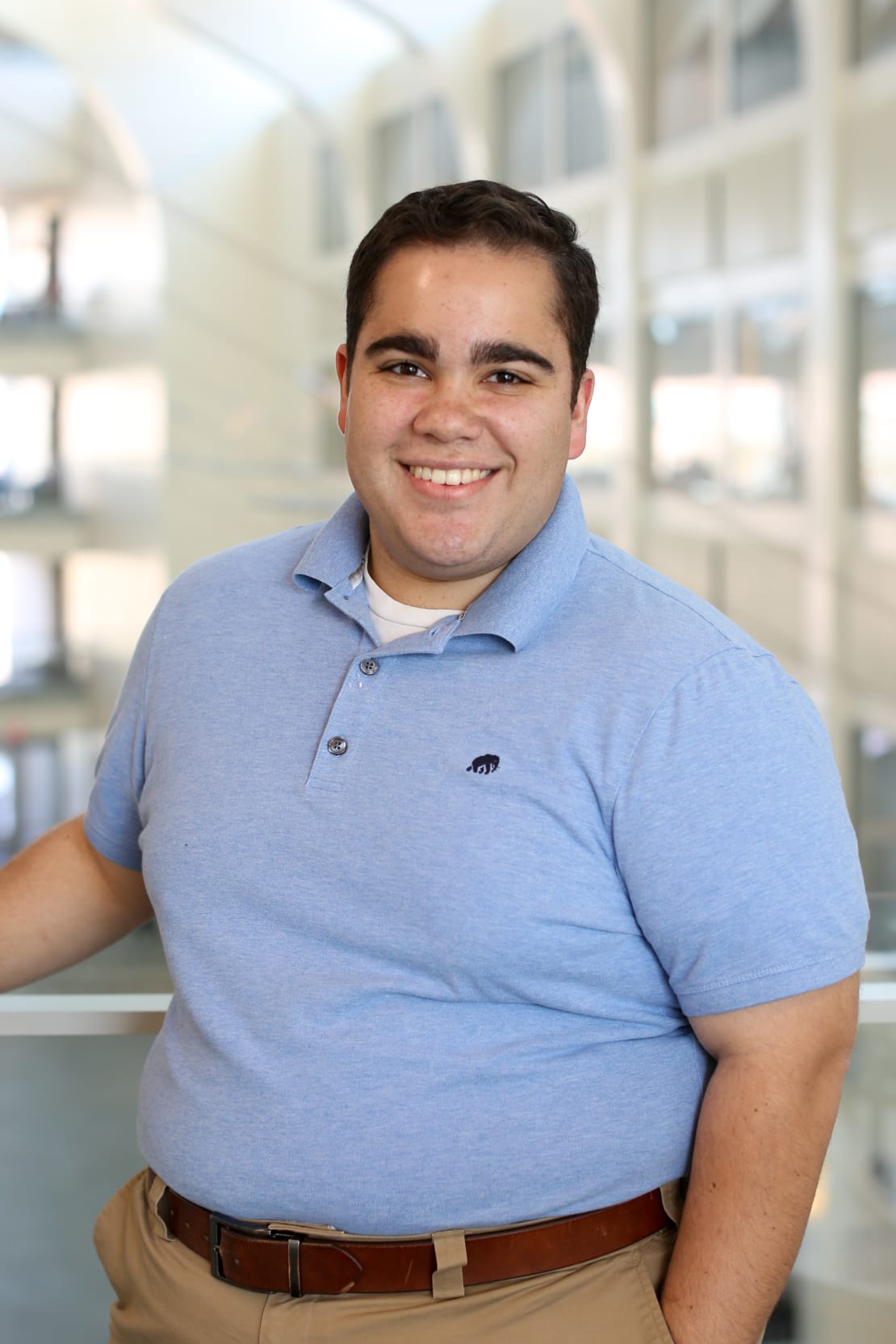
Using a novel method developed by Maximilian Meier, an assistant professor at Chiba University in Japan and colead on the study, they were able to identify how “clumpy” or stochastic an event was, which was helpful because true neutrino events are more stochastic than the cosmic-ray background.
“The non-observation of cosmogenic neutrinos tells us, under some pretty conservative modeling assumptions, that the cosmic-ray flux is mostly composed of elements heavier than protons,” says Brian Clark, an assistant professor at the University of Maryland and colead on the study. “This is a big open question and something scientists have been trying to answer for almost one hundred years.”
Clark adds that the two other large-scale particle astrophysics experiments—the Pierre Auger Observatory and the Telescope Array—have been trying to answer the same question for almost a decade. Because they measure the cosmic-ray air showers directly, interpreting the data relies on sophisticated modeling of the nuclear physics of cosmic-ray interactions. This is where IceCube offers a complementary approach that, as described in the paper, is largely insensitive to those modeling uncertainties. This makes it an important, independent confirmation of the results obtained by air shower experiments.
“This is the first time a neutrino telescope has managed to do this. And it was a major promise of the discipline, so it’s very exciting to see it happen,” says Clark.
Future studies by the IceCube Collaboration will look to machine learning in order to extract the most out of the IceCube data.
“We are really excited to see the next generation of detectors, like IceCube-Gen2, come online, which will be ten times larger than IceCube and, therefore, significantly increase our capabilities to detect cosmogenic neutrinos in the future,” says Meier.
+ info “A search for extremely-high-energy neutrinos and first constraints on the ultra-high-energy cosmic-ray proton fraction with IceCube,” IceCube Collaboration: R. Abbasi et al. Submitted to Physical Review Letters. arxiv.org/abs/2502.01963