The search for cosmic sources of high-energy processes in our Universe requires large-scale observatories. IceCube, the cubic-kilometer neutrino detector at the South Pole, is only one of them. Large-scale gravitational-wave (GW) observatories, like LIGO in the US or Virgo in Europe, use km-scale interferometers to search for space-time ripples that propagate like a wave. These gravitational waves were predicted by Albert Einstein almost one hundred years ago and might be produced by sources such as binary systems of neutron stars or black holes, which might also be producing high-energy neutrinos.
Multimessenger astronomy analyses are very interesting for cosmic source searches since they can bring information on both the origin of high-energy emission and the dynamics and structure of the process driving the emission. GWs, for example, produced by the bulk motion of the source, carry information on the dynamics of its central region. High-energy neutrinos, on the other hand, require hadron acceleration in, for example, the relativistic outflows from this central generator.
In a joint analysis by the IceCube, LIGO and Virgo collaborations to be submitted to the journal Physical Review D, researchers aimed to identify GW events and high-energy neutrinos that could originate from the same astrophysical source and to determine their joint significance. Data taken during coincident runs between 2007 and 2011, when IceCube was running in its 22-, 59- and 79-string configurations, was analyzed. No significant coincident events were found, but the search allowed researchers to derive upper limits on the rate of joint sources for a range of source emission parameters.
This research covers practically all the coincident data taking periods between initial LIGO-Virgo GW detectors and IceCube and, thus, constitutes a very comprehensive dataset preceding the start of advanced GW detectors expected in 2015 for LIGO and in 2016 for Virgo.
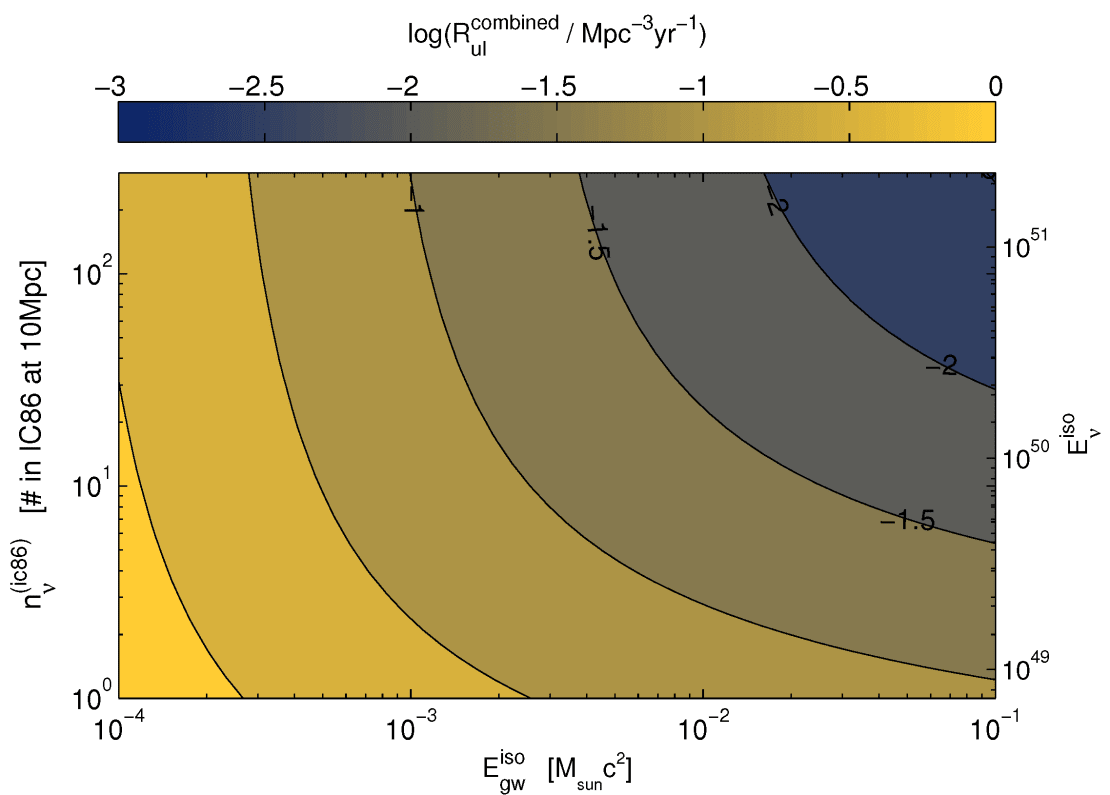
The use of more than one cosmic messenger may also reduce the false alarm rate, that is, the frequency of occurrence of events from background alone, with a greater significance. Requiring temporal and directional coincidence from a joint event is an effective filter in reducing the background and is beyond the identification capabilities of single-messenger searches. This will be particularly important for the first GW discoveries.
“I am intrigued by the opportunity to use the two most elusive messengers to learn about the most energetic cosmic processes in a way that was not possible even a few years ago. Among so many scientific avenues, it is particularly interesting to look for invisible cosmic explosions, making use of the fact that both gravitational waves and neutrinos can escape from dense environments where photons are trapped. It also helps that gravitational-wave and neutrino detectors continually observe practically the entire sky, so we can look for cosmic events without needing to point our telescopes in the right direction,” explains Imre Bartos, a LIGO researcher at Columbia University, who co-leads the joint effort of LIGO/Virgo and IceCube.
“The beauty of the multimessenger search is that the coincidence of timing and direction can help us zero in on a single neutrino event that would otherwise have gone unnoticed in a sea of background events. Working together with the LIGO/Virgo experiments, we all increase the chances of discovering something new,” adds Chad Finley, an IceCube researcher at Stockholm University, who also co-leads the collaboration of IceCube with the LIGO/Virgo experiments.
About LIGO and Virgo
LIGO has two Michelson interferometers in the US, one in Hanford (Washington) and one in Livingston (Louisiana), both with 4-km arm length. LIGO detectors are sensitive to GWs in the frequency band of 80-7000 Hz. Virgo is a Michelson interferometer with 3-km arm length located near Cascina, Italy, and it’s sensitive to GWs in a frequency band similar to that of LIGO.
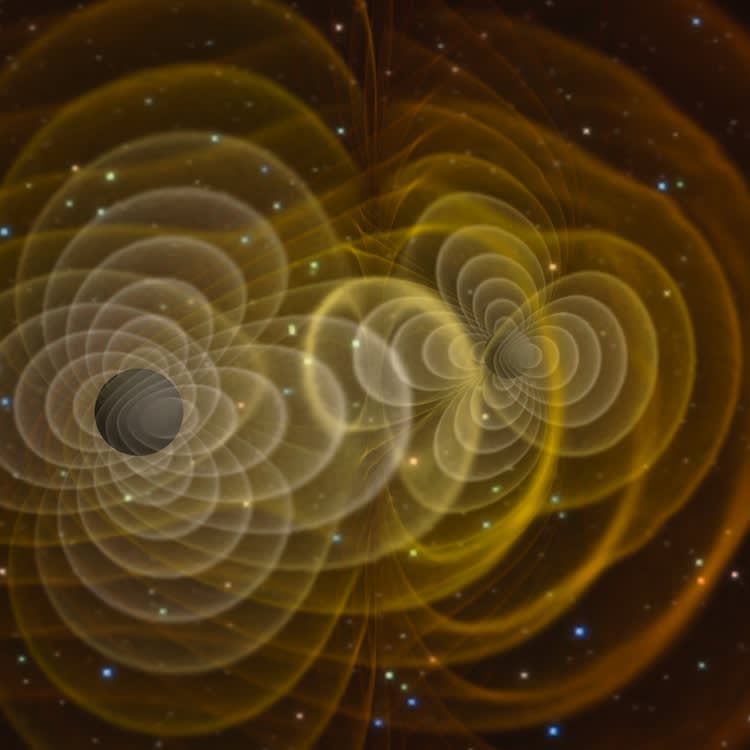
The three instruments detect GWs by monitoring the relative displacement of test masses (mirrors) placed at the end of the interferometer arms. An incoming GW will change the distance between the test masses with an amount proportional to their arm length. In each interferometer, a prestabilized laser light is emitted that later splits to each arm. So, if a gravitational wave passes through the detector, modifying the space-time in the local area, it can be detected as a phase difference between the two laser beams.
There are a multiple sources that can emit both GWs and high-energy neutrinos, such as those producing gamma-ray bursts (GRBs) or soft-gamma repeaters (SGRs). GRBs have two main progenitors: massive stellar core collapses with rapidly rotating cores, and neutron star-neutron star and neutron star-black hole mergers. Neutrinos would be more abundantly produced in the first scenario, while GWs would be more copious in the second one. SGRs are thought to be produced by highly magnetized neutron stars.
The horizon distance for GRBs, which is the maximum distance at which the GW signal from an optimally oriented and optimally located source can be detected, reached tens of megaparsecs for the initial LIGO-Virgo detectors. For SGRs, the most optimistic scenarios only reached sources within the galaxy.
Info Read the story on LIGO’s site.
Info “Multimessenger Search for Sources of Gravitational Waves and High-Energy Neutrinos: Results for Initial LIGO-Virgo and IceCube,” The IceCube Collaboration, The LIGO Scientific Collaboration, The Virgo Collaboration. Physical Review D90 (2014) 10, journals.aps.org arXiv.org:1407.1042